3 ECS Key Application Areas

3.5
Agrifood And Natural Resources
In 2022, the conditions of the planet changed as abruptly as foreseen by the Intergovernmental Panel on Climate Change (IPCC). According to the latest report from IPCC 192, global warming is causing major changes in precipitation patterns, oceans and winds, in all regions of the world and, in some cases, irreversibly so. The intensification of natural phenomena is putting the viability of life on Earth at risk, and from now on will have serious repercussions on food security, health, and sustainable development. Extreme hot temperatures in normally cold countries; melting of the poles at an accelerated rate, and consequently, rising sea levels threatening coastal areas; prolonged droughts in previously fertile and productive places on different continents; scarcity of fresh and affordable water for human consumption in large cities are just some of the issues that we are now facing. Moreover, forest fires have doubled worldwide in the last 20 years destroying around 3 million hectares each year particularly in boreal forests, leaving the largest climate change related carbon deposits on the planet so far according to a study conducted jointly by three institutions: Global Forest Watch (GFW), World Resources Institute (WRI) and the University of Maryland (UMD). The study concludes that we will lose or degrade these important lungs in the medium term despite these forests being one of our best defences against climate change.
There is a strict relationship between climate change and agriculture. The two-way relationship of climate change and agriculture is of great significance because we need to adopt effective practices to mitigate risks to human health and crop production.
On one hand, as explained above, impact of climate change on agriculture is mainly related to extreme heat events and reductions in precipitation and water availability, that are harming, as a result crop productivity On the another hand, the contribution of agricultural activities to climate change is related to two specific factors:
- Farming in particular releases significant amounts of greenhouse-gas emissions, in particular methane and nitrous oxide.
- Agrochemicals released to fight against pests contaminate soils and waters as a direct consequence of the use of these substances. However, climate change is only one of many problems that agriculture must face193. In fact, growing global demand and competition for resources, food production and consumption need to be redesigned in a proper way, linking agriculture, energy, and food security.
Consequently, contemporary economic and ecological challenges mean our food production must support a new balance between production in quantity and production of quality. Achievement of this new balance in food production exposes us to risk in various forms (war, market fluctuations, large scale public health and animal health) including climate variability. These risks must be immediately addressed to obtain a transition towards a more sustainable and inclusive food system from farm to fork.
As a primary sector, agriculture is not a trivial sector to be totally digitalized because of the great variability (e.g., climate or other natural phenomena) of events on crops and lands. Moreover, the farmers will need to change mentality. Service support and maintenance with an adequate education on the new technologies must be introduced, as well as a robust and reliable precision farming infrastructure. All of this creates lots of opportunities for the ECS community to contribute to the disruption of the agrifood sector. Innovation and digitalization of agriculture are becoming more and more relevant due to the need for building a new level of agri-food system resilience, capable of having a more productive and sustainable agriculture globally.
Smart Internet of Things (IoT) systems have become very important for the sustainable production and consumption of safe and healthy food, as well as for sustainable practices in agriculture, livestock, aquaculture, fisheries, and forestry. They can also foster access to clean water, fertile soil and healthy air for all, in addition to helping preserve biodiversity and restore the planet’s ecosystems. In short, the use of these connected objects (IoT) helps the stakeholder to increase productivity while ensuring sustainability.
In this Chapter, five Major challenges have been identified. The first two Major Challenges relate to livestock and crop health, connected to farming systems and food supply chain assurance and management. For instance, IoT system technologies can be used in pest management or towards minimising the use of pesticides, fertilisers and antibiotics. Farming systems and food supply chain management benefit from smart IoT systems, including the use of traceability framework with trustworthy and security features194, as well as from robots and drones, to revolutionise modern agriculture and food production. The third Major Challenge addresses issues such as soil health, air quality and the environment, all in terms of smart integrated monitoring technologies, and the use of smart waste management systems and remediation methodologies. The objective is to protect the environment to reduce the destruction of ecosystems caused by a myriad of anthropogenic activities and reduce GHG emissions. The fourth Major Challenge refers to the key role that IoT systems can play in water quality monitoring and access to clean water. An important aspect here is the overall management of water usage, as well as smart treatments to foster the circular use of wastewater, rainwater and storms/floods. The fifth Major Challenge relates to biodiversity restoration for ecosystem resilience, how electronic components and systems (ECS) can contribute to the restoration/preservation of a greater variety of crops, and greater fauna and flora species diversity, to ensure the natural sustainability of healthy ecosystems (agriculture, aquaculture, fisheries and forestry) by enabling them to better withstand and recover from misuse, abuse or disasters.
All five Major Challenges in this Chapter align with key Horizon Europe missions, as well as the European Green Deal and existing and emerging digitalisation technologies. To efficiently address these challenges, significant advances are crucial in the fields of new materials, manufacturing technologies, information, and communications technology (ICT), Artificial Intelligence (AI), robotics, electronics and photonics, and other technologies, as well as in circular industries. Figure F.79 illustrates the shifts on technology and demand that will be required to have a more resource efficient consumption together with an open-source sustainability.
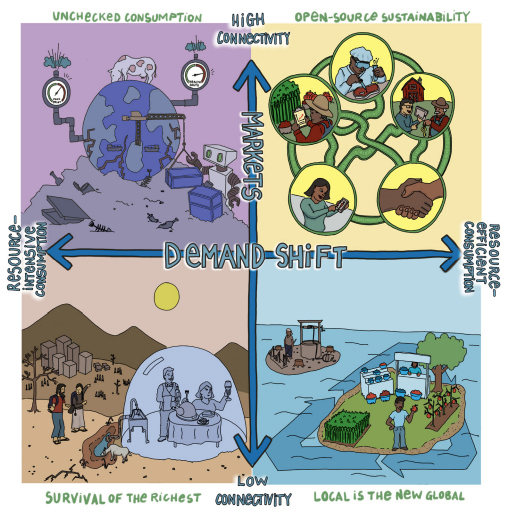
External requirements
According to the UN, if the global population reaches an expected 9.6 billion by 2050, the equivalent of almost three Earth planets could be required to provide the natural resources needed to sustain current lifestyles. Increasing food production is driven not only by population growth, but also by more demanding and sophisticated diets as populations become wealthier. On the other hand, productivity is being hit hard by climate change in regions where food scarcity and inefficient resource management is most prevalent. The necessary acceleration in productivity growth is being hampered by the degradation of natural resources, a reduction in biodiversity, and the spread of transboundary pests and diseases of plants and animals, some of which are becoming resistant to antimicrobials196. Investments in changing agricultural practices and incorporating technological innovations has boosted productivity, but the yield growth is far from sufficient. A more holistic and innovative approach is needed to reduce the strain on natural resources and enhance their quality, while also increasing food productivity. At the same time, food losses and waste claim a significant proportion of agricultural output, whereas poor bio-waste management and packaging increases environmental pollution.
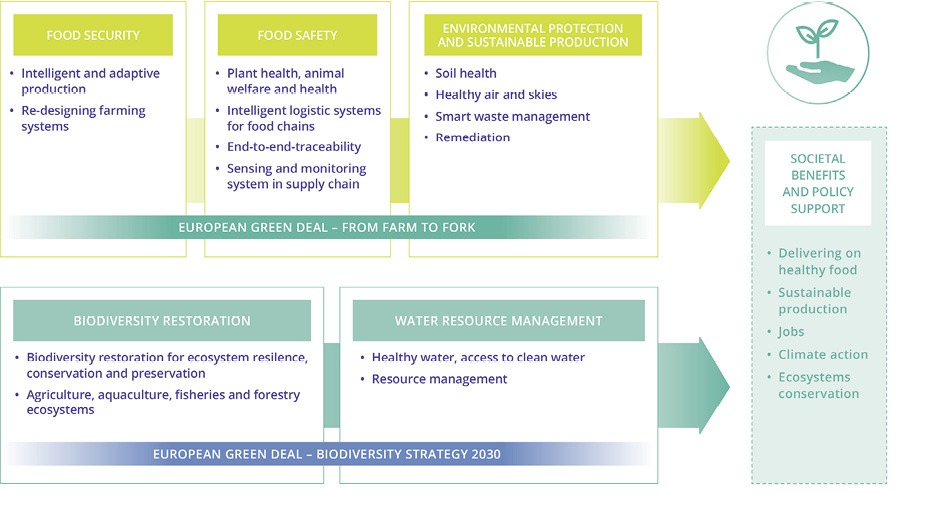
Addressing key issues on food security and sustainable production would lessen the need for production increases while improving the natural resource base. For example, mitigating the effect of natural and human pressures on water bodies is a priority, namely by reducing general pollution and plastics, eutrophication, acidification and warming up as much as possible. Less than 2.5% of the world’s water is fresh197, and water pollution in rivers and lakes is occurring faster than nature can recycle and purify. Currently, more than 2 billion people live with the risk of reduced access to freshwater resources198, and by 2050 at least one in four people is likely to live in a country affected by chronic or recurring shortages of freshwater. Now, 2.6 billion people are economically dependent on agriculture199 despite 52% of arable land being moderately or severely affected by soil degradation. Air quality has also been deteriorating in both rural and urban areas because of the spread of particulate matter in addition to the release of greenhouse gases (GHGs) all of which have detrimental effects on the population200 and on the climate.
Today, farmers still spread much more fertiliser than is required on their fields. Consequently, excess nutrients such as nitrates and phosphates accumulate in the soil and filter into groundwater with a dramatic impact on the environment and public health. Therefore, there is increasing pressure on the agricultural sector to find sustainable solutions for reducing environmental pollution caused by fertilisation. Smart production processes and intelligent logistic systems across the whole supply chain are some of the solutions that can yield optimisations to reduce or at least to keep emissions constant with increased productivity, while ensuring safe food production. This is particularly important given that currently, every year, almost one in 10 people will fall ill due to food-borne diseases201.
The pandemic crisis has shown the vulnerability of the overall agri-food supply chain when compromised by employee illness or travel restrictions enforced by the lockdown constraints. These circumstances appear unprecedented, but they are relevant for every type of pandemic that could occur worldwide, crossing international borders and affecting large populations.
New epidemiological methods that utilize dynamic network analysis202 to analyse the key drivers of emerging pathogen movement are needed. In the agrifood sector, this is not more renounceable because the overall agrifood elements are strictly interconnected (from seeds and plants to livestock management and crop production, as well as postharvest transportation are single point of risks of pathogen and mycotoxin movement in stored food).
Effective surveillance strategies are essential to support agrifood health programs, crisis prevention and improvement in biosecurity. Therefore, ECS solutions are the efficient way to contribute in all this.
Societal Benefits
In response to an ever-increasing set of challenges faced by the world, the UN defined 17 Sustainable Development Goals to act as a blueprint for achieving a better and more sustainable future for all. The SDG implementation plans (SDG 2, 6, 12 and 15 are particularly relevants) to address the global challenges we face in protecting biodiversity, our natural resources and acting on climate change. Furthermore, it includes actions relating to socioeconomic drivers aimed at eliminating poverty, hunger, inequality, and achieving responsible consumption and production, sustainable prosperity, peace and justice. In Europe, national and EU policies such as the “From Farm to Fork”203 and “Biodiversity Strategy 2030”204, reflect and amplify the underlying SDG objectives with a set of measures – from regulatory frameworks to incentives and investments for development, and the deployment of holistic innovative approaches in a circular economy, agroecology, agroforestry, climate smart and sustainable agriculture, bioeconomy and the Blue Economy.
Within the Horizon Europe framework programme on research and innovation, it is envisioned that Europe will make significant progress with respect to high-impact missions on: “adaptation to climate change including societal transformation”, “cancer”, “healthy oceans, seas, coastal and inland waters”, “climate-neutral and smart cities” and “soil health and food”.
Innovative solutions based on IoT systems have a significant socioeconomic impact for the EU in rural, coastal and urban areas. For instance, agriculture is being transformed by the IoT revolution, with the use of reliable sensors and smart devices, as well as cost-effective and secure interoperable IT systems allowing farmers to better control the process of raising livestock and growing crops. As a result, safety and quality in food production are rapidly evolving, becoming more predictable and more efficient than ever. According to the European Innovation Partnership “Agricultural Productivity and Sustainability” (EIP-AGRI)205, the digitalisation of rural areas can help to improve the economic and environmental sustainability of the agricultural sector. Moreover, it can make farming more attractive for young people, improve the quality of life of farmers and multiply the number of rural businesses. Consequently, rural depopulation could be greatly reduced. ,For instance, the Smart Water Management (SWM) project206 points to an acceleration in the deployment of smart water networks with the aim of upgrading the reliability, efficiency, quality control, sustainability and resilience of drinking water supply services while also educating end-users on the benefits of water conservation. Strategies of this nature could represent the solution for urbanisation-related issues (scarcity, pollution, etc.) by providing a better use of our water resources while protecting the most vulnerable places, and by creating innovative types of economy and management.
Developments in smart IoT systems for agriculture and food production based on innovative and advanced ECS will strongly contribute to reaching the objectives set by the European Green Deal and the following three main actions and respective targets:
1. From Farm to Fork
Moving towards a fair healthy and environmentally friendly EU food system by 2030 through the targets listed in Topic 1207.
2. Natural resources
Topic 2: Targets set for natural resources.
3. EU Biodiversity Strategy for 2030
Topic 3: Targets set in Biodiversity Strategy for 2030208.
Topic 1: From Farm to Fork |
Target actions 1.1: Reduce the use of pesticides in agriculture that contribute to pollution of soil, water and air |
|
Target actions 1.2: The excess of nutrients in the environment as a major source of air, soil and water pollution, negatively impacting biodiversity and climate |
|
|
Target actions 1.3: Antimicrobial resistance linked to the use of antimicrobials in animal and human health leads to an estimated 33,000 human deaths in the EU each year |
|
|
Target actions 1.4: Organic farming as an environmentally friendly practice that needs to be further developed in the EU each year |
|
|
Topic 2: Natural resources |
Target actions 2.1: Optimisation and remediation towards climate-neutrality – first step for 2030 and then 2050, through |
|
Topic 3: EU Biodiversity Strategy for 2030 |
Target actions 3.1: Establish protected areas |
|
Target actions 3.2: Restore degraded ecosystems at land and sea across the whole of Europe |
|
F81 - Targets set in the Farm to Fork strategy, in Natural resources and EU Biodiversity Strategy for 2030.
Figure F.82 depicts the agrifood value chain and the main actors involved, along with a list of the benefits obtained for farmers and consumers by using smart IoT systems. Moreover, the advanced technology applied throughout the whole chain will bring new market opportunities for the European semiconductor and ECS industries.
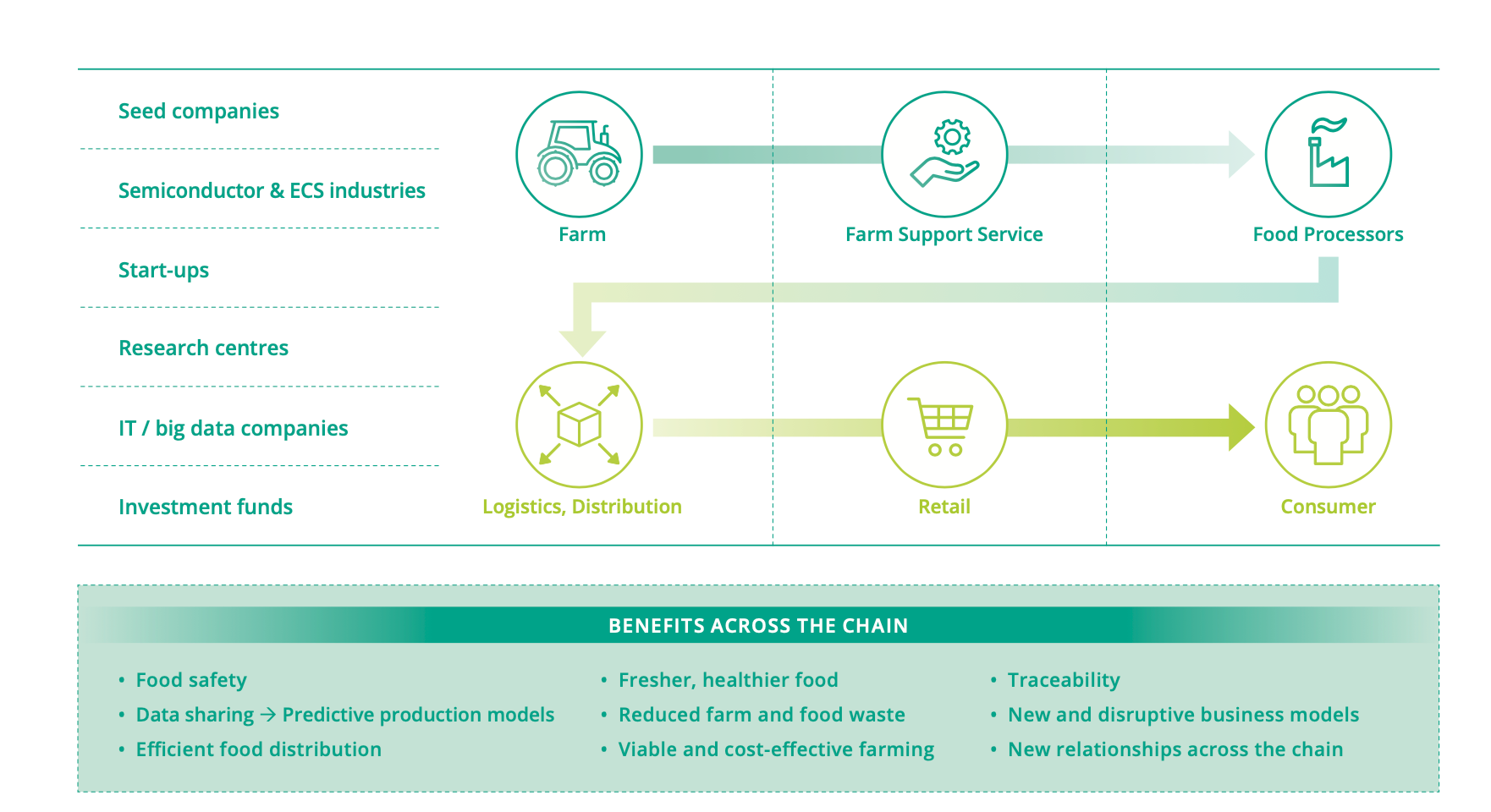
This section discusses the five Major Challenges that need to be addressed in the domain of agriculture (food security, food safety, environmental protection, and sustainable production), natural resources and biodiversity, and how smart IoT systems and associated key enabling technologies can help achieve them.
- Major Challenge 1: Food Security.
- Major Challenge 2: Food Safety.
- Major Challenge 3: Environmental protection and sustainable production.
- Major Challenge 4: Water resource management.
- Major Challenge 5: Biodiversity restoration for ecosystems resilience, conservation, and preservation.
3.5.4.1 Major Challenge 1: Food Security
Food security209 and food safety210 are two complementary/interdependent concepts that are characterized in different ways: one indicates the economic and social security of availability of food supplies; the other indicates their health and hygiene safety. All together these terms refer to the same processes linked to agricultural production, and it is relevant to manage them simultaneously.
Figure F.83 presents the interrelation between food security and foot safety concepts, as well as their main constituent elements. This section and the next will address the challenges related to food security and food safety from an ECS perspective.
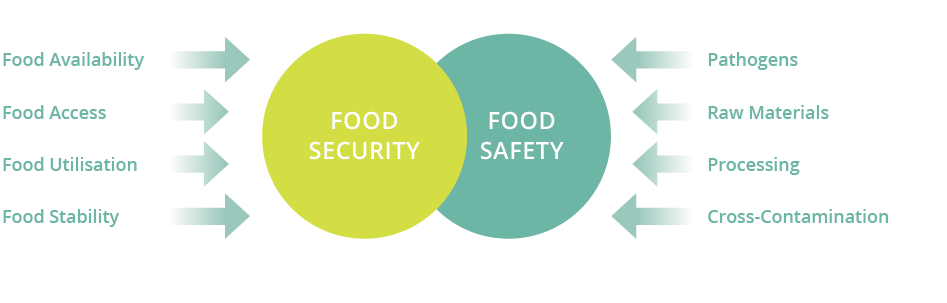
Consolidated advances in Industrial Internet of Things (IIoT) have already started to shape smart manufacturing in the food and beverage211 industry. Access to relevant and role-based information, in real-time or near real-time, is key to ensuring the efficient storage and processing of data, and their appropriate use for optimised decision-making at every level of next-generation automation systems and robotics, e.g., cyber-physical systems (CPS). Therefore, sustainable production, safety and quality do not only depend on the product itself, they also depend on respective processes and their control as offered by key data gathering and monitoring, smart sensing, data analysis, diagnostics, and control systems. Ultimately, intelligent food production frameworks can consider consumer needs in specific markets, and such systems can provide intelligent recommendations for adjusting the amount and quality of food accordingly, assuring food security (i.e. enough food for each market) and food safety (i.e. healthy food), while also considering environmental concerns and societal impact.
Following the trend in manufacturing industries, digital twins212 are the next step for the food industry and farming systems. In short, digital twins allow digital/virtual representations (models) of physical objects and processes, coupled with behaviour models that enable simulation and prediction upon changes to variables associated with the objects or the surrounding conditions. Digital twins are remotely and real-time connected to the objects in the physical world to reflect the dynamics of real systems. Thus, digital twins are expected to take the farming and food industry to the next level in terms of productivity and sustainability. Essentially, they will become tools for ultimate optimisation of decision-making by integrating the variables from the production process and the variables from the market and consumers. In any case, development of digital twins in farming is far behind its counterpart in the manufacturing industry for several reasons including because physical objects in farming are “living” objects (crops, trees, animals…), and because variables of interest in farming are highly heterogeneous and are complex to model and measure.
To develop intelligent food production systems, solutions are required in (but not limited to) the following fields:
- In-line inspection, networked packaging systems and robot technology in the warehouse to allow for a smart workflow to manage, monitor, optimise and automate all processes accordingly.
- Intelligent control room systems to enable correlations between machine malfunctions and load parameters to be detected immediately, thereby enabling maintenance work to be carried out early and on schedule, with a reduction in costly downtimes.
- Food industry imposes specific requirements (e.g., in food processing) that may take advantage of smart bio-sensing high-quality monitoring to reduce the amount of water and chemicals used in such processes, and to prevent contamination.
- AI/machine learning (ML) and big data models must be devised and used to offer further intelligent decision-making and, whenever possible, should be employed directly at-the-edge for greater energy efficiency.
- IIoT systems, based on AI and digital twin technology, can provide the flexibility to tailor-make new products to help cope with ever demanding diets.
Precision Farming Systems
Advanced farming machines and robotic collaborative systems are needed for cost-effective land and livestock management, as well as for large-scale arable and fruit crops management. Tasks can be performed in parallel, enabling economies of scale. Advanced machines include the following:
- Harvesting robotic systems: autonomous robots or swarms of light(er) robots (causing less soil compaction) can replace intensive and strenuous labour practices as the worldwide population transitions from rural to urban areas and manual labour declines as farmers get older. Agricultural robots need to be equipped with improved capabilities for sensing and perception. Special attention must be paid to safety and trustworthiness aspects for those robots expected to work collaboratively with humans or close to livestock.
- Drones: remotely piloted or autonomous unmanned aerial vehicles (UAVs), either flying alone or in swarms, can mainly improve efficiency in two application areas: (i) monitoring large areas with intelligent computer vision devices to provide a higher level of detail and on-demand images, especially as drones can overcome limitations of satellite imagery (e.g., images below forest cover); and (ii) in the use of phytosanitary (plant health) products to increase efficiency and reduce environmental impact by avoiding indiscriminate chemical dispersion and following predetermined prescription maps.
- Satellites: these allow for improved information regarding fields, although a combination of data from further sensors with increased update frequency, improved performance and spatial resolution would also be needed. Moreover, small satellites (micro, nano) could provide larger IoT connectivity services when Internet coverage may not be available at any location where a WSN network should be deployed.
- Wireless sensor networks (WSNs) and smart actuators deployed across fields will form the backbone of heterogeneous - multi-agent - collaborative approaches. Local parameters (e.g., ambient temperature, soil pH, soil salinity, relative humidity, etc.) measured from multiple sensors planted in the soil or attached to the plants could be retrieved remotely by drones and/or robotic systems to deepen the field analysis provided by image-based techniques. Coupled to the proper AI and decision systems, WSNs will also further help in automatically triggering the appropriate actions (e.g., drones could locally release agrochemicals after interrogating/analysing sensors, water irrigation systems could be activated only in some land areas, etc.).
- Digital farming Support as a Service (FAAS).
- Most of the farms in Europe are small scale, below 10 hectares, whilst only about 1% of all farms are above 500 hectares 213. An important challenge remains that all sizes of farms, including small and medium sized, should have access to digital solutions, namely cost-effective ones, and to facilities to easily exploit them. Whilst large scale farms have the means to setup and maintain large infrastructures and even robotic appliances, smart scale farms should benefit from the provision of digital support solutions, for instance, farm-monitoring via sensors, experience sharing, education, remote assistance, local maintenance, virtual cooperation, precision agriculture applications, etc. - as a service. This kind of services should be created and provided through local cooperatives or new service providers specialised in the HW infrastructure and SW applications involving several types of expertise in agronomy, communication, data analytics, computer science, etc. Having digital farming as a service, business models may help to finance CAPEX while technology transition to provide better cost model scenarios and facilitate the deployment of this technology in the European farms.
Couplings214 between the technologies cited above and the data sources are possible and make it possible to enrich knowledge and respond to other field issues. Take the example of the drones above. Georeferenced imagery data by drones can be completed:
- On wider perimeters but with lower frequencies by satellite imagery.
- By images acquired by proximity sensors onboard self-propelled vehicles (tractors or robots) and their associated equipment during interventions in the plots as well as during pedestrian observation phases by farmers.
- By data collected via networks of communicating sensors covered by the Connectivity chapter.
All the proposed solutions should meet important requirements such as cost-efficiency, compactness, reliability, lifetime, low power, security, interoperability with existing machinery and between systems implementing appropriate security schemes and taking human factor. into account. Furthermore, training systems based on virtual, augmented, and mixed reality and simulators are needed for training people (e.g., operators), independent of seasonality or safety issues. However, an important challenge remains that all sizes of farms, including small and medium sized, should have access to these solutions, namely cost-effective ones, and to facilities to easily exploit them.
Horticulture/greenhouses, urban and vertical indoor agriculture
Urban agriculture is being promoted as a promising option for sustainable food, a better quality of life, and community engagement. The goal of this modern version of agriculture is to grow and deliver high-quality food with a minimal waste of resources.
Many crops in vertical indoor farms are often cultivated using hydroponics, a technique where there is no need for soil and fertiliser as the growing plants are supplied with irrigation water. In fact, recent environmental challenges have promoted the intensification of “soil-less agriculture” in an urban context to decrease the negative impact on nature. Even if hydroponics produces quality crops with high efficiency, there is an area of opportunity here to better monitor and control the fertiliser components in the irrigation water, such as through the development of:
- Novel and low-cost online sensors for optimised control, such as nutrient sensors to enable smaller discharge of fertiliser into natural waters.
- Robots with a high precision level to perform automatic harvesting to reduce the overall production costs, which are currently high, to be competitive with traditional agriculture.
- Autonomous indoor farming systems in which cultivation is controlled remotely via AI, based on measurements of crop properties with the help of intelligent sensors and AI-based digital twin models of such plants.
3.5.4.2 Major Challenge 2: Food Safety
Application of high-tech sensors and AI to monitor, quantify and understand individual plants and animals, as well as their variability, to ensure food safety is key for next generation novel ecology-based agricultural systems. Smart sensors and monitoring technology that can adapt to the unpredictability and variation of living systems are required. This Major Challenge will require integrated digital technology solutions such as ecology-based robotic systems that can control the bio-physical processes (including growing conditions) and understand the biological environment (for plants and animals). However, innovative ecology-based robotic systems’ manipulation of operations is a huge challenge in environments that are only modestly defined and structured. Furthermore, detection in the supply chain and “at the fork” should be also considered. This implies low-cost compact sensors, connected to information processing systems used in the food supply chain and by consumers, that allow, for instance, freshness and food safety detection for meat and vegetables (which could be integrated into smartphones).
Integrated pest management (IPM)
Novel IPM strategies are needed to detect diseases and prevent their spread on crop production for European organic and conventional agriculture, and to increase organic farming and horticultural systems. Improved IPM will require developments in the following fields:
- Smart systems based on portable real-time pest disease diagnostics and monitoring platforms to provide rapid local and regional disease incidence alerts (georeferenced) e.g., weather/climate information for predictive models providing risk assessments and decision support for IPM.
- IoT devices specialized in pests and disease measurements, such as insect traps and other systems based on image recognition or AI models.
Agro ecology based: Move from conventional to organic, regenerative agriculture
To support the EU “From Farm to Fork” implementation, smart ECS can help farmers to drastically decrease the use of pesticides and heir impact on human health and the environment. This will require:
- Development of cost-effective and intelligent intra-row, herbicide-free weeding techniques using advanced robots and robot fleets for individual plant recognition with high precision, based on advanced (vision) sensor technologies and AI algorithms working under in-field conditions.
- Development of smart and power efficient sensors to monitor the quality of spraying, as this is essential for biocontrol products and contact pesticides.
- Integration, into the same framework, of decision-support tools and precision agriculture tools to simplify farm management, improve crop quality and reduce costs.
- Dedicated services such as technical support (infrastructure deployment and maintenance), precision agriculture as a service, education, etc.
Plant precision breeding and plan phenotyping
The development of smart technologies can support precision plant breeding and phenotyping. This could be nanotechnology solutions or smart sensor solutions to support the following:
- Genomics and transcriptomics: DNA informed breeding, gene editing, genome prediction, breeding optimisation, phenotyping and seed sowing optimization.
- Large scale and high precision measurements of plant growth, architecture and composition: these measurements are required to optimize plant breeding by increasing our understanding of the genetic control and response of plants to their environment. Sensor systems should allow the study of plants in relation to biotic and abiotic factors, including plant-microbiome interactions, plant-plant competition, plant diseases and exposure to a multitude of variable abiotic environmental conditions such as light quality, irradiance levels, nutrient supply, temperature, humidity, soil pH and atmospheric CO2 levels.
Livestock health is crucial for food safety. Different animal pathogens can be a serious threat for livestock management, because pathogens can be divided in those that can infect multiple species, and those that infect specific terrestrial and aquatic animal species (i.e., cattle, sheep, goats, equines, etc.). A great threat is represented by diseases that they are highly transmissible, having the potential to spread rapidly across borders and cause significant socio-economic and public health consequences. All these lead to great economic loss to the farmers. Moreover, the use of antibiotics to treat animals increases the cost of production, as well as creates other problems like residues and resistance, which is of serious concern to public health.
In addition, best livestock management practices must reduce the release of pathogens into the environment and substantially reduce the risk of microbial contamination of surface and groundwater. As outlined in the literature215, there is relevant impact of animal pathogens in four areas: animal health, economics, food safety and security, and public health. Consequently, there is strong interest in developing new advanced solutions systems with high sensitivity and specificity, for early detection of animal diseases and minimizing antibiotics use too.
Synergistic strategies can be applied to approach these challenges. Agronomic and technological solutions must be applied to:
- Minimise risks (e.g., release of pathogens into the environment, use of antibiotics for animals).
- Surveillance of the livestock environment through an efficient process of digitalization of Livestock management to prevent diseases spread.
From a microbiological perspective, microorganisms can mitigate risk because specific beneficial microorganisms can be selected to work synergistically with other microorganisms already in the environment. Beneficial microorganisms can support the nutritional requirements of plants and reduce the incidence of pathogenic microorganisms, to solubilize minerals, to conserve energy, to maintain the microbial-ecological balance of the soil, to increase photosynthetic efficiency, and to fix biological nitrogen.
Research has also studied beneficial effects when these specific microorganisms are included in animal diets216. It has been shown that when they meet the organic matter that makes up the animal’s diet, they secrete beneficial substances such as vitamins, organic acids, chelated minerals, and antioxidants, influencing an antibacterial effect through a selective blocking of pathogen colonization.
Animal welfare is also an important concern for a growing number of consumers.
We can assess that these synergistic strategies (risk reduction with agronomic solutions and surveillance) as explained above are drivers for investing in better sensing systems for animal monitoring. Combined with data analytics solutions, this will improve animal health and welfare, resulting in more animal-friendly production, higher efficiency, better quality, and improved food control safety:
- Wearable sensors at the farm/barn level, and ambient sensors during cattle transport.
- Smart sensor systems to monitor animal activity, such as individual or group behaviour, to provide useful information for early detection of diseases and to increase animal well-being.
- Smart sensor systems for rapid verification of bacterial infection together with behavioural observations to control disease spread and support clinical and veterinary stakeholders to effect suitable therapeutic interventions; body temperature can also be monitored for early disease detection to reduce antibiotics use.
Intelligent logistic systems including sensing and monitoring for food chains
Logistics are a critical component of the food chain. They not only determine the reach of distribution, but logistics delays and conditions profoundly affect the quality and safety of products reaching consumers, and can result in food loss and waste in the supply chain.
Smart real-time sensing, monitoring and control systems in the food supply chain will safeguard food quality and food safety, while eventually reducing food losses in the supply chain. Therefore, technological solutions are required, but not limited to:
- Systems for monitoring and controlling the quality of food products and ingredients during transport and storage (e.g. temperature in cold chain monitoring, moisture, controlled atmosphere, ethanol, ethylene), which should be reliable, secure, efficient and interoperable along the logistics chain.
- Predictive systems to assess quality of (perishable) food products in the supply chain, providing real-time decision-support based on actual sensor measurements, supply chain data and AI models.
- Transport route optimisation, considering not only time and cost, but also external conditions and the intrinsic properties of the products being transported.
These needs are strongly related with traceability, as shown in the following section.
Food and beverage manufacturers and producers are faced with increasingly complex and fragmented supply chains, stricter regulation, and more demanding consumers. Regulatory compliance, competitive advantage, brand reputation and costs have made product traceability a priority and end-to-end traceability a major challenge. In today’s globalised world where people of any origin live across every country, the source of food products and ingredients, as well as their certification, are a major concern and priority for consumers, who want to be sure about the origin of food products. Therefore, traceability should also encompass certifying food origin, as well as making information available on any relevant process to which the food product has been submitted. This information should not be restricted to mere tracking across the supply chain. End-to-end traceability solutions are required, but should not be limited to:
- Integrating blockchain into current technology to increase safety while preventing fraud and counterfeiting.
- Traceability to increase alignment between production and individual consumer demands, leading to better provisioning and more personalised nutrition support.
- Traceability to optimise distance between farm and fork – although many products are produced preferentially in specific parts of the world, there are also many examples of food that could be produced economically closer to consumers.
- Smart tags.
To this end, as IoT/IIoT solutions are increasingly being deployed, integrated hardware systems need to deliver (apart from mobility and connectivity) long lifetime autonomous sensing and AI-based intelligence at-the-edge, as well as edge and/or cloud analytics and cybersecurity, complying with privacy regulations when applicable, on a plug-and-play, open, interoperable architecture, and platform.
Distributed Ledger Technologies (DLTs) such as blockchain allow secure storage and tracking of all kinds of information, including condensed sensing or monitoring data regarding crops and livestock. Examples include information on crop seeds, feed ingested by livestock (including medication and antibiotics), as well as recording of the whole process that any farm product is submitted to until it reaches the consumer, throughout the respective supply chain and involved actors. Such information increases the transparency of these supply chains and can reduce potential production issues, e.g., simplify the tracing of eventual product spoiling, or other issues, to the respective source, supporting possible decisions to recall product batches if necessary. Consumers can also benefit from such transparency, i.e., they can be given access to information to make better informed decisions about the offered goods they want or need to acquire, and they can use the information to provide feedback to farmers and producers incentivising further their policies and practices.
Nowadays, end-consumers are more concerned about the origin of the agriculture products that they consume. A complete system to manage traceability and to offer, to the end-consumers, a complete transparency of the actions taken in the farm (for instance in the vineyard) and during the transformation process (in the example of a winery) is required: a solution could be using blockchain. However, it is important that small-scale farmers with low technological expertise, resources, and insufficient size to integrate blockchain could be supported, eventually through a dedicated ecosystem. This can be devised through blockchains implemented to include such farmers and respective food product chains, which would also allow supporting and further assuring food safety, namely in respective local markets, eventually involving less costs associated with logistics and distribution, and thus also contributing to sustainability and fair food systems.
Nevertheless, the lack of regulations, standardization and interoperability are challenges for incorporating blockchain.
3.5.4.3 Major Challenge 3: Environmental protection and sustainable production
EU regulations together with consumers’ increased interest in organic food, is compelling farmers to drastically decrease the use of pesticides to reduce risks and impact on human health and the environment, as well as to undercut the maximum residue levels of pesticides. Pesticides are found not only in drinking water217 but also in food and beverages. Lively debates have shown that our society demands alternatives to pesticides to help preserve the environment and improve food quality.
Drastic reduction in the use of pesticides is one of the major goals of the EU’s agricultural policy, with some countries planning to halve their pesticide use by 2025 (e.g., ecophyto plans218 in France, and the Aktionsplan Pflanzenschutzmittel219 in Switzerland). The EU Farm to Fork strategy also aims to implement an action plan that significantly reduces risks from chemical pesticides, as well as the use of fertilisers and antibiotics, and to increase the amount of organic farming carried out in Europe.
In general, the new EU agriculture policies put a major focus on preservation of landscapes, biodiversity, and environmental protection, in a results-oriented model aligned with the Green Deal.220 For instance, the reform of the Common Agriculture Policy (CAP) introduces measures for fostering the adoption of sustainable farming practices (“eco-schemes”), such as agroecology or organic farming. Farmers will need to provide “digital evidence” of compliance to the CAP rules and the implementation of good practices. The CAP evaluations will be largely based on the use of high-quality data collected directly from the field. This will require measurement and monitoring technology (for environmental performance, biodiversity monitoring parameters, etc.) which is accurate, highly scalable, and secure (certified monitoring information).
Areas of interest are often remote without sufficient connectivity – new approaches are required to flexibly deploy sensors and collect data.
The in-situ real-time monitoring of soil nutrients and herbicides
The optimal use of chemical fertilisers and organic manures to deliver the required increase in food production requires a complete understanding of applied nitrogen- and phosphorous-based nutrients with a much greater spatial and temporal resolution than is available today. Current methods of soil analysis do not provide real-time in situ nutrient analysis in fine detail, and delays in receiving soil results are common because of backlogs in commercial labs due to high sample volumes, thus reducing the value of the soil test results for the farmer. Moreover, herbicides are another huge problem due to their environmental and health impact. To solve these issues, the following actions must be done:
- Intelligent sensors (with miniaturised and ultra-low power consumption components) must be developed to deliver measurements of soil nutrients in situ and in real time at parts per million (ppm) concentrations. Such devices must have the appropriate packaging to extract water from the soil. Ideally, they should be buried in the soil for long periods of time or at least while sustaining operation capabilities for the entire growing season. To optimise effectiveness, low proximity sensors should be combined with optical sensors and high proximity sensors to retrieve the maximum amount of information on soil health.
- Likewise, smart actuators could prove to be highly beneficial. Such miniature units could be deposited on or buried into the soil. Coupled to sensing functionalities into the same module, critical actions (e.g., release of agrochemicals) could be triggered very close to the plant roots for maximized efficiency.
- Multidisciplinary approaches for developing novel sustainable smart ECS are needed. Indeed, current ECS contain a variety of toxic materials and chemicals. As such, they cannot be left in the soil. The optimization in the use of agrochemicals should not come at the expense of another ecological burden. New sustainable "green" ECS made of eco-friendly materials that will have benign environmental impact must be created.
- IoT systems with edge and/or cloud-based data analytics are also necessary to provide farmers with decision-support regarding fertilisation strategies, by translating measurements into meaningful agronomic indicators and respective measures. These strategies should prioritise the use of organic fertilisers and the gradual reduction of chemical fertilisers until eliminated to restore the biodiversity contribution in the preservation of soil health. Furthermore, this type of system should detect weeds, preserve the “good ones” and eradicate those that are competing with the crop in question. This requires low-cost vision technologies (not only plain optical red/green/blue (RGB), but also 3D, hyperspectral imaging, etc.) and edge AI for in situ prompt recognition.
Sensors and diagnostics for air quality monitoring (indoor, urban and rural)
According to the World Health Organization, the air we breathe is growing dangerously polluted. Nine out of ten people now breathe polluted air, which kills seven million people every year. There has been much progress on identifying and reducing the sources of air pollution at lower concentrations and with higher spatial coverage. This is necessary to provide adequate data on what people are breathing, and to provide localised as well as holistic solutions. Microsensors and/or mini-stations can be used during fieldwork campaigns in cities, but there are technical problems relating to power source, data transmission, data storage, and data handling and assessment. Besides, local measures are not always effective since local concentrations of particulate matter may be influenced by long-range transported pollutants from agricultural activities occurring outside city boundaries.
Similarly, while indoor air quality has been shown to unambiguously impact the wellness, health and performance of people as shown for instance with the Covid-19 in the schools by lack of indoor air quality measurement. There is also a lack of spatial granularity and a significant lag between exposures and sensing, actuation and management interventions for risk mitigation. In addition to indoors, air quality is made more complex by the interaction between indoor and outdoor air, emissions from buildings and their contents (paints, furniture, heating, and cooling systems, etc.), human activities (breathing, cooking, cleaning, etc.) and the effects of long-term exposure to low concentrations of volatile organic compounds. These issues necessitate the development and deployment of real-time intelligent multi-sensor technologies with high selectivity and embedded (re-)calibration techniques. These should be combined with a monitoring network (edge-based) as part of the indoor infrastructure to provide the spatial and temporal information needed for specific, targeted and appropriate actions. Such actions should also include public awareness and the promotion of behavioural changes.
Smart systems for controlling and preventing GHG emissions
Strong evidence has been accumulated on the climate emergency resulting from human activities that add GHGs to the Earth’s atmosphere. The EU is the world's third biggest GHG emitter after China and the US. Although several measures have been taken since the Paris Agreement, breakthrough technologies and state-of-the-art deployment are still needed across the transport sector and other industries with a high emission footprint to achieve a further reduction in emissions. These would be facilitated by the following:
- Smart systems and digitalisation to improve industrial processes performance and energy/resource efficiency towards a low-carbon economy, while reducing the impact of mobility and agricultural processes on the environment and human health, thereby controlling and preventing GHG emissions.
- A focus on the GHG emissions from animals by investigating microbiological sensing technologies on or in animals (in their rumens, for instance) to increase efficiency while reducing environmental impact, as well as performing analysis of the gathered data to support decision-making for mitigation measures (for instance, leading to change in feed).
Integrated bio-waste systems
Despite proactive European policies and regulations221, effective bio-waste management remains a challenge. Reducing, recycling and reusing food/kitchen waste requires significant progress in technological solutions along with strong policymaking and shifting community behaviour. These solutions could be based on the following:
- Smart monitoring, controlling waste treatment units in real-time as well as gas emissions in landfills and anaerobic digestion monitoring. Data analytics should include gamification for behavioural triggers.
- Smart waste collection bins (radio-frequency identification (RFID) tags, self-compacting bins, fullness level sensors, automated waste segregation), including automated robotic systems and optimised separation systems, which can be complemented by the upcycling of waste streams into usable resources and optimal routing systems, as well as vehicle tracking. These solutions should be integrated and interconnected into the product life cycle “from cradle to grave” to enable circular and resource-efficient methodologies.
Intelligent sustainable / biodegradable packaging
Intelligent and biodegradable packaging concepts have been gaining traction in the food industry to improve product safety and reduce environmental impact. Smart sensors in an IoT system can monitor environmental conditions and product quality, while communication devices can store and convey data throughout the product life cycle. While these concepts need to be further advanced for efficient, safe food production and waste management, intelligent packaging itself needs to become more sustainable. Novel ideas are required to solve the problem of the amount of plastic packaging produced by food manufacturers. The definition of biodegradable packaging should lead to a new generation of food packaging. Such novel ideas include:
- A synergetic interdisciplinary approach to cross the boundaries of novel materials for food packaging and smart sensors associated with analytical methods for the detection of harmful substances that can infiltrate into food, cause water contamination, etc.
- Fabrication and hybrid integration of eco-friendly nanostructured electrodes, sensors, energy harvesting and storage devices on rigid and flexible biodegradable substrates to reduce the waste from embedded electronics in smart packaging.
Efficient smart networks for remediation
Remediation processes aimed at converting harmful molecules into benign ones can be undertaken in different ecosystems, such as water bodies (e.g., biotic, and abiotic farming by-products), air (e.g., GHGs) and soil (e.g., pesticides). Remediation processes are mainly carried out in wastewater treatment plants. Although some pollution sources are static and sufficiently well known such that treatment can be undertaken effectively, other pollution sources are more mobile in both time and/or space, making treatment at single points unsatisfactory. Another limiting issue is that remediation technologies are often power-intensive and cannot be deployed for long in remote locations. Alternative high-efficiency remediation methods are needed, such as to transform/reduce the levels of CO2 in chemical products. Current devices are also prone to fouling. This means remediation processes cannot be run constantly in remote locations, and there is thus a necessity to undertake them only when and where they are most required. In this regard:
- A network of smart sensors (an IoT system) that can monitor relevant status in real time, and inform on the necessity of remediation, would provide unique decision support invaluable for efficient water, air, and soil management.
- Techniques used in the measurement and analysis of carbon sequestration by soils could also investigate the current potential of soils as a remediation mechanism to improve the sequestration capacity – such investigation should include the initiative of “four per 1000”222 presented at COP21 in Paris.
- Likewise, tools and methods able to evaluate the performance of the carbon sequestration techniques employed should be developed to guarantee their efficiency.
3.5.4.4 Major Challenge 4: Water resource management
The quality of groundwater, surface water bodies (oceans, seas, lakes), waterways (rivers, canals, estuaries) and coastal areas has a great impact on both biodiversity and the quality of water that people consume every day. While natural droughts may lead to increased salinity in freshwater systems223 and along with floods, impact or endanger the quality of water bodies, human activities in energy production, manufacturing and farming industries have a major detrimental effect through thermal pollution, chemical, microbiological and micro-plastic contaminants, and biotic and abiotic farming by-products. Moreover, the outdated and deteriorating water infrastructure is having a detrimental impact on both water quality and the amount of water lost through leakage.
In the context of climate change, increased water temperatures may cause (apart from extreme evaporation) eutrophication and excess algal growth in surface water bodies. Moreover, heavy storms may increase the amount of sediment nutrients in water sources, which have a direct impact on drinking water quality. Therefore, climate change jeopardizes the quality and safety of our water, making the development of new tools to deal with this problem more critical than ever.
Healthy Water
With the aim of reducing pollution-related problems, water utilities, water associations, academia and private industry have focused on developing new methods, policies and procedures to secure drinking water distribution by (1) detecting in real-time any compound, contaminant or anomaly that may represent a health risk for the end-users and (2) taking the required measures to mitigate these issues. This necessitates online information on the status of water sources at a larger scale than ever before. To mitigate both accidental and intentional contamination of freshwater resources, the deployment of sensors and diagnostic systems with rapid communication technologies and data analysis capabilities are needed to secure water quality and its distribution over the network. Such actions would provide:
- Connected and highly integrated multi-parameter diagnostic sensors for real-time physico-chemical analysis (temperature, ionic electrical conductivity, pH, turbidity, inorganic pollutants as nitrates or heavy metals, etc.) in water distribution network and wastewater treatment plants, and biofilm growth monitoring in water pipes.
- Online monitoring systems at the edge, including devices with embedded AI for data analysis. The presence of a mesh of intelligent devices in drinking water networks will make it possible to identify and deal very quickly with drifts or anomalies (e.g, leaks, contamination events, etc.) while reducing the amount of data sent to the servers.
Integrated systems for demand reduction and conservation of water
According to the UN Development Programme224, dwindling drinking water supplies affecting every continent. On the one hand, increased urbanisation and farming have amplified the demand of water for human consumption and for domestic and agricultural use. On the other hand, an increasing number of countries are experiencing water stress due to longer drought periods and the spread of desertification. In addition, approximately 25% of all urban drinking water is being lost forever225 in global water systems, before it even reaches the end-user. Therefore, there is an urgent need to prevent losses from water abstraction as climate effects intensify. Leak localisation is currently very time-consuming, labour-intensive, and costly. Operators must manually place equipment that “listens” to the water flow during the night. Smart integrated systems can significantly contribute to key measures aiming at affecting consumer practices in water usage, delivering greater efficiency in detecting leaks and reducing water waste. Developments are needed in the fields of:
- Smart metering, time-of-use pricing and gamification to control consumption and appliances, along with interoperable solutions for a truly connected smart household (taps, lavatories, showers, appliances).
- Low-cost sensors for flow control, leak detection and auto shut-off, along with inexpensive actuators to remotely control valves for limiting water usage by volume/time. IoT systems can optimise the control of household, agricultural and industrial infrastructure/equipment in water-intensive processes.
- Smart systems able to automate leak localisation, and to respond promptly and cost-effectively. This can be a combination of in-pipe inspection (to locate the leak) and a network of low-cost, fine-grained sensors to allow predictive maintenance of distribution systems.
Efficient and intelligent water distribution
The main challenge for improving the use of water is to guide its distribution depending on its final application (drinking water, water for industry, etc.). However, the existing sanitary regulations always look to optimise water safety regardless of its final use. To apply the most effective measures to make water distribution more efficient, it is necessary to thoroughly review the different supply protocols and quality criteria for each sector. Moreover, by continuously monitoring the quality and availability of water, it would be possible to better regulate its distribution depending on the final use and to adjust the price accordingly. Intelligent systems connected to smart grids will allow water inputs to be made in the network at the right times, optimising the energy cost as a result.
To address these challenges, there is a need for developing:
- Novel smart metering solutions based on various technologies, including electrochemical multi-parameter sensors (pH, chlorine, conductivity, etc.) with high stability, anti-fouling, high accuracy capabilities and cost-effectiveness, as well as optical sensors based on different principles (fluorescence, absorbance, etc.) integrated into miniaturised systems at a low cost.
- Robust IoT systems with adequate data analysis processing power and AI capabilities to handle the large volume of data generated by the different water management processes to satisfy quality, usage type and associated pricing.
- Efficient year-round water management in terms of storage to deal with some of the most urgent shortages, with better forecasting and warning systems based on extensive measurements – e.g., intentionally flooded areas could be used to store water in times of expected scarcity.
Smart systems for irrigation management
At a global level, agriculture consumes 69% of the world’s freshwater226. Because of this, precise control of irrigation is essential to guarantee water and food security for all. Irrigation water management is the practice of monitoring and managing the rate, volume, and timing of water applications according to seasonal crop needs, considering the soil intake and water holding capacities with the objective of using water in the most profitable way at sustainable production levels. To this end:
- Smart sensors are increasingly required as tools to implement irrigation management and monitor water levels. Sensors should be more intelligent to support real-time applications and/ or reduce latency, optimise power consumption of the overall system, and facilitate local (at the edge) cost-effective solutions for both outdoor and indoor use.
- Integration of systems monitoring water deficiency or surplus is also required. These could be based on narrow-band spectral reflectance of water and land surfaces for vegetation/habitat mapping, along with UAV utilisation in remote areas.
Smart systems for flood management
Flood management has been gradually integrating smart sensors. IoT systems with water-level sensors can also play a significant role in real-time monitoring and natural hazards predictive/forecasting capacity models. This requires:
- The monitoring of water levels and devising prediction models to identify areas at a high risk of flooding. This is possible through the development and deployment of more intelligent sensors in combination with smart predictive algorithms to integrate information from other sources, such as weather forecasts and regional georeferenced data.
- IoT interoperable systems are key for provision of real-time information to first responders, civilians and companies to proactively take countermeasures.
Smart water treatments fostering circular use (wastewater, rainwater, storm water)
Around 80% of all wastewaters is currently being discharged into the world’s waterways, where it creates health, environmental and climate-related problems. Water from industrial, agriculture and domestic use contains organics, phosphates, nitrogen, cellulose, rare earth elements and other substances. In addition to its domestic use, purifying, distilling, or deionising water is essential for many agricultural and industrial uses – to ensure the consistency of products and to meet strict safety regulations. The global market for water and wastewater technologies reached US $64.4 billion in 2018 and is expected to rise to US $83 billion by 2023227. Technologies that allow resource recovery from wastewater to be commercially feasible are increasingly being developed, making transitioning to a circular economy an opportunity to accelerate and scale-up the most recent scientific and technological advances that support greater efficiency in the water sector. There is an increasing requirement for:
- A range of sensors in water systems to monitor water levels, the flow of water through different channels, temperature changes, chemical leakage, pressure level, chemical residues, etc.
- IoT-enabled water purifiers that can predict potential system failures to reduce downtime in public water systems, and to enable remote sensing for mapping groundwater resources and monitoring sustainable extraction levels.
3.5.4.5 Major Challenge 5: Biodiversity restoration for ecosystems resilience, conservation, and preservation
It has been stated that: “Biodiversity boosts ecosystem productivity where each species no matter how small, all have an important role to play"228. For example, increasing the number of plant species means a greater ensuring natural sustainability for all life forms229. Healthy ecosystems can better withstand and recover from a variety of disasters, anthropogenic or not. Healthy biodiversity offers many natural services for everyone.
It should be noted that there are many such services that we already get for free! However, the cost of replacing these, even, if possible, would be extremely expensive. More than ever, as noted in Section 3.5.4.3, the new EU agriculture policies promote sustainable farming practices that help to protect the environment, preserving landscapes and biodiversity. This is a consequence of the well-recognized correlation between the health of ecosystems and the health of farming production. It therefore makes economic and development sense to move towards sustainability. From this perspective, ECS will contribute to addressing some of the key challenges relating to biodiversity and sustainability for the four ecosystems described below.
Agriculture is one of the economic activities that has the highest dependence on nature and biodiversity230. On average, global mean crop yields of rice, maize and wheat are projected to decrease between 3% and 10% per Celsius degree of warming above historical levels. All crops depend directly on soil health and fertility, and more than 75% of global food crop types rely on animal pollination. However, the impact of agriculture activity on the environment must be as low as possible to preserve biodiversity.
In this regard, the EU Biodiversity Strategy 2030 establishes several objectives231, summarized in sub-section 3.5.5 Timeline. To address these objectives, there is a need to develop:
Aquaculture impacts biodiversity negatively in several ways232: (i) where antibiotics and hormones are used to reduce farm stock mortality and improve growth rates, but their use has side effects for the flora and fauna of water bodies receiving farm effluents; (ii) through eutrophication and changes in flora and fauna in waters receiving effluents from aquaculture facilities; (iii) through the risk of excessive exploitation of wild fish stocks for use in farm fish feeds; and (iv) by transfer of disease and parasites from farm animals to wild animals.
To address these side effects, there is a need to develop:
- Precision aquaculture systems for optimal feeding (minimizing waste and feed residuals), optimal use of antibiotics/hormones, and optimal use of freshwater.
- Smart multi-sensors and smart systems for monitoring water quality in aquaculture facilities and their effluents.
- Smart systems combining data collected from different sources (IoT networks, satellite, and drones) and data analysis based on AI/ML techniques to create predictive models leading to more confident decision-making, timely alerts, and automated systems in general.
The EU’s Biodiversity Strategy has set an objective of protecting a minimum of 30% of its sea area. Like agriculture, fishing is an economic activity with a strong dependence on biodiversity. Keeping fish stocks healthy is critical to guaranteeing ocean biodiversity and thus the economic sustainability of fisheries. According to recent studies, the preservation of marine stocks could increase the annual profits of the European seafood industry by more than €49 billion.
Fishing activities impact biodiversity negatively in several ways, particularly by: (i) increasing fish mortality, so measures must be taken to keep this under maximum sustainable yield levels; and (ii) damaging the ocean ecosystem due to the use of certain fishing techniques, currently the most damaging activity to the seabed. In addition, the effect of by-catching from non-selective industrial fishing methods endangers many species of marine animals not being fished for. It is therefore necessary to evolve towards more selective and less damaging fishing techniques, as well as the more effective control of illegal fishing practices.
To reduce these negative impacts, there is a need to develop:
- Oceanographic sensing and monitoring solutions (including unmanned vehicles, UXVs) for fisheries ecosystems to estimate biodiversity indices, fish stocks and species distribution, and to build fishery management systems consistent with conservation objectives and rules.
- Technologies to make fishing gear more selective and environmentally respectful.
- Technologies for checking compliance and detecting illegal activities (onboard cameras, RFID, traceability technologies, vessel monitoring, etc.).
The EU Biodiversity Strategy has set the objective of protecting a minimum of 30% of the EU's land area. At least one-third of protected areas - representing 10% of EU land - should be strictly protected. In particular, the strategy identifies the crucial need to strictly protect all the EU's primary and old-growth forests, which are the richest forest ecosystems removing carbon from the atmosphere, while storing significant carbon stocks. The strategy also calls for preserving the good health and increasing the resilience of all EU forests, especially against wildfires, droughts, pests and diseases. It is envisaged that the European Commission will develop a forest information system for Europe that integrates data from multiple sources and providers. To prevent more wildfires, we need to grow rural economies in a sustainable way and manage climate change, so a much better understanding and continuous assessment of EU forests is necessary. To this end, there is a need to develop:
- A precision forestry system with remote sensing and Al/ML monitoring capabilities to map and assess the condition of the EU forests as well as early detection and prevention of threats to the forests (wildfires, pests, diseases, etc.).
- Smart systems for environment monitoring of forests and fields as well as CO2 footprint monitoring, remote monitoring of wildlife behaviour and habitat changes, and provide timely warning on illegal poaching activity.
- Customised services, not only to support the above-mentioned systems but also to further exploit the information they provide.
The IoT system technologies and related activities prioritised in this Chapter are pillars to addressing the specific challenges of food, agriculture and natural resources. The Major Challenges of food safety and security, environmental protection and sustainable production, water resource management and biodiversity restoration are significant to leverage the future of agriculture through agrifood innovation and interdisciplinary collaboration. They are aligned with the European missions on ”adaptation to climate change including societal transformation”, “healthy oceans, seas, coastal and inland waters”, “climate-neutral and smart cities” and “soil health and food”. The application needs under those missions can be addressed through the integration of robust smart IoT systems together with innovative technological solutions, as well as holistic approaches in processes covering the whole supply chain, from resource utilisation and production to food packaging, waste management and remediation. As such, there are potential synergies with the Health, Energy, Mobility and Digital Industry application chapters.
Destination Earth (DestinE)233 is an EU flagship initiative that aims to build a digital model of the Earth “to monitor and predict the interaction between natural phenomena and human activities”, as part of the global Green Deal strategy. Two of the three main components of the DestinE system, namely the Data Lake and the Digital Twin, can benefit from the advances in IoT technologies proposed in this chapter. The data lakes will need to be fed from a large variety of data sources, including of course the data captured in the field by IoT sensors. Likewise, the digital twins will need to integrate comprehensive IoT data from farms, forests and oceans. On the technology side, the envisaged IoT system solutions will require significant advances in terms of functionality. Synergetic topics include advanced multi-sensing and data fusion capabilities, energy autonomy (harvesting, storage, and power management), connectivity, interoperability at all levels, lifecycle properties, reliability, privacy and security. There are also great challenges to make these heterogeneous systems manufacturable at the right cost for market entry while simultaneously achieving miniaturisation, ultra-low-power consumption, adequate packaging considering the environmental conditions and other constraints. To this end, significant collaborative effort will be required in materials integration and process technologies, architecture and embedded software for individual components, devices, systems and systems-of-systems. New designs at different abstraction levels (component, devices, network) and computing (edge, cloud) paradigms, new and interoperable models (e.g., AI/ML/DL and digital twins), design flows and methodologies and their associated tools are also needed. This is required to cross boundaries between domains, e.g., for verification and automated design space exploration, as well as data analytics and decision-making through AI/ML/DL and digital twins-based solutions. Overall, an orchestrated synergetic approach in the areas of advanced materials, circular industries, manufacturing technologies, ICT, AI, digital twins, edge and cloud computing, robotics, electronics, and photonics, as well as other key technologies such as new advanced HMI like virtual, augmented and mixed reality, will facilitate technology- push/demand-pull advances in the development and exploitation of smart IoT agriculture and natural resources.
192 IPCC Intergovernmental panel on climate change,Climate change Mitigation of climate change - https://www.ipcc.ch/report/ar6/wg3/downloads/report/IPCC_AR6_WGIII_Full_Report.pdf
193 Agriculture and Climate change, European Environment Agency, 30 June 2015 - https://www.eea.europa.eu/signals/signals-2015/articles/agriculture-and-climate-change
194 K. Demestichas, N. Peppes and T. Alexakis. "Survey on security threats in agricultural IoT and smart farming." Sensors 20.22 (2020): 6458.
195 Shaping the Future of Global Food Systems: A Scenarios Analysis, World Economic Forum White Paper 2017
196 [FAO UN: The Future of Food and Agriculture, 2017]
197 All about water, https://www.iaea.org/sites/default/files/publications/magazines/bulletin/bull53-1/53105911720.pdf
198 UN 2019 The Sustainable Development Goals Report 2019: Goal 6: Clean water and sanitation / https://unstats.un.org/sdgs/report/2019/The-Sustainable-Development-Goals-Report-2019.pdf
199 ibid: Goal 15
201 Food safety - World Health Organisation at https://www.who.int/news-room/fact-sheets/detail/food-safety
202 The persistent threat of emerging plant disease pandemics to global food security at https://doi.org/10.1073/pnas.2022239118
203 https://ec.europa.eu/food/sites/food/files/safety/docs/f2f_action-plan_2020_strategy-info_en.pdf
205 https://ec.europa.eu/eip/agriculture/en/publications/eip-agri-seminar-multi-level-strategies-digitising-0
208 Facsheet EU-Biodiversity-strategy-en.pdf, https://ec.europa.eu/commission/presscorner/detail/en/fs_20_906
209 Food security has been defined by the FAO as “Food security exists when all people, at all times, have physical and economic access to sufficient, safe and nutritious food that meets their dietary needs and food preferences for an active and healthy life”
210 Food safety is an umbrella term that encompasses many facets of handling, preparation, and storage of food to prevent illness and injury. Included under the umbrella are chemical, microphysical and microbiological aspects of food safety
211 Beverage will be considered as food in the rest of the document.
213 Destatis Statistisches Bundesamt, Betriebsgrößenstruktur landwirtschaftlicher Betriebe nach Bundesländern, retrieved October, 6, 2022 from https://www.destatis.de/DE/Themen/Branchen-Unternehmen/Landwirtschaft-Forstwirtschaft-Fischerei/Landwirtschaftliche-Betriebe/Tabellen/betriebsgroessenstruktur-landwirtschaftliche-betriebe.html
214 See in French https://blog.irt-systemx.fr/ameliorer-la-production-du-secteur-agricole-avec-les-nouvelles-solutions-technologiques/
215 T. A. McAllister, E. Topp, Animal Frontiers, Volume 2, Issue 2, April 2012, Pages 17–27, https://doi.org/10.2527/af.2012-0039
216 D. Hidalgo, F. Corona & J.M. Martín-Marroquín Biomass Conversion and Biorefinery volume 12, pages 4649–4664 (2022)
217 https://www.notre-environnement.gouv.fr/themes/sante/la-pollution-de-l-eau-douce-ressources/article/les-pesticides-dans-les-eaux-souterraines?lien-ressource=5193&ancreretour=lireplus
218 Ministère de l’Agriculture, Le Plan Ecophyto, qu’est-ce que c’est? https://agriculture.gouv.fr/le-plan-ecophyto-quest-ce-que-cest , 2020
219 Aktionsplan Pflanzenschutzmittel, https://www.blw.admin.ch/blw/de/home/nachhaltige-produktion/pflanzenschutz/aktionsplan.html
220 https://aioti.eu/wp-content/uploads/2022/02/AIOTI-Role-of-IoT-in-addressing-agroecological-focus-of-Green-Deal-Final.pdf
221 https://www.consilium.europa.eu/en/press/press-releases/2018/05/22/waste-management-and-recycling-council-adopts-new-rules/
222 Researchers from the French National Institute for Agronomic Research, mentors of the project, have observed that by increasing the organic matter in the soil by 4 grams per 1000 - hence its name - it would be possible to limit the current growth of CO2 emissions to the atmosphere. Promoting good agricultural practice would combat climate change and, at the same time, guarantee the food security of the population.
225 Leakage Reduction in European Water Mains; Layman Report https://ec.europa.eu/environment/eco-innovation/projects/sites/eco-innovation-projects/files/projects/ documents/-curapipe_layman_report.pdf
227 https://www.bccresearch.com/market-research/environment/water-and-wastewater-treatment-technologies-global-markets.html
228 Anup Shah « Why is biodiversity important? Who cares? https://www.globalissues.org/article/170/why-is-biodiversity-important-who-cares
230 European Commission. The business case for biodiversity. May 2020. https://ec.europa.eu/commission/presscorner/detail/en/fs_20_907
231 European Commission. EU Biodiversity Strategy for 2030: bringing nature back into our lives. May 2020. https://eur-lex.europa.eu/legal-content/EN/TXT/?qid=1590574123338&uri=CELEX%3A52020DC0380
232 Claude E. Boyd, What is biodiversity and its relevance to aquaculture certification? https://www.aquaculturealliance.org/advocate/biodiversity-relevance-aquaculture-certification/