3 ECS Key Application Areas

3.2
Energy
3.2.1.1 Change towards the carbon neutral society and challenges for ECS
Energy systems supplying clean, affordable and secure energy are the focus of The European Green Deal. To achieve this goal, the European Union set targets for a renewable energy share of 32 percent and a Greenhouse gas emission reduction of 55 percent by 2030. Renewable energies bring several benefits such as mitigating climate change, emission reduction as well as improvements in the European energy security153. Although the EU even surpassed its’ 2020 target of 20 percent, sustained action with an accelerated pace is necessary to prepare the economy and society for the upcoming climate challenges. The drop in CO2 emissions was overcome rapidly by the uneven recovery from the Covid-induced recession (Figure F.53), which put a major strain on the European energy system with a rebound in coal and oil use154. The power sector must further be transformed from fossil fuel based to renewable generation and, at the same time, needs to grow in order to enable decarbonization of mobility, industry, and thermal energy supply and reach the climate targets. The recent change in the supply strategy in Europe to be independent of strategically critical gas or oil suppliers is a further boost for renewable energies and efficiency measures. The shortage of materials (e.g. batteries and other electronic equipment) has already had a serious effect on the R&D and direction of developments. Some materials are getting rarer or can contribute to conflicts. Furthermore, there is a growing shortage of skilled workers, which is a huge societal challenge and needs to be compensated by fast technological progress and innovation. Therefore, smarter components are needed to compensate the growing shortage of technical knowledge and skills.
Because of the increasing residual load resulting from the local mismatch between decentralized renewable generation and load, a digitally controlled transmission and distribution infrastructure is required. Thus, electronic components and systems (ECS) are key to future energy systems being optimized in both, design and operation, for high efficiency, low CO2-emissions, cost, and security of supply. The development of energy systems is driven by action against climate change, booming decentralized renewable generation (solar, wind), digitalization and AI technologies, as well as cyber security issues. The Energy Chapter highlights the Major Challenges in the changing energy landscape based on electrical energy generation, supply, conversion, and use. Highest efficiencies and highly reliable, secure solutions are required to achieve the change towards a carbon neutral society in 2050.
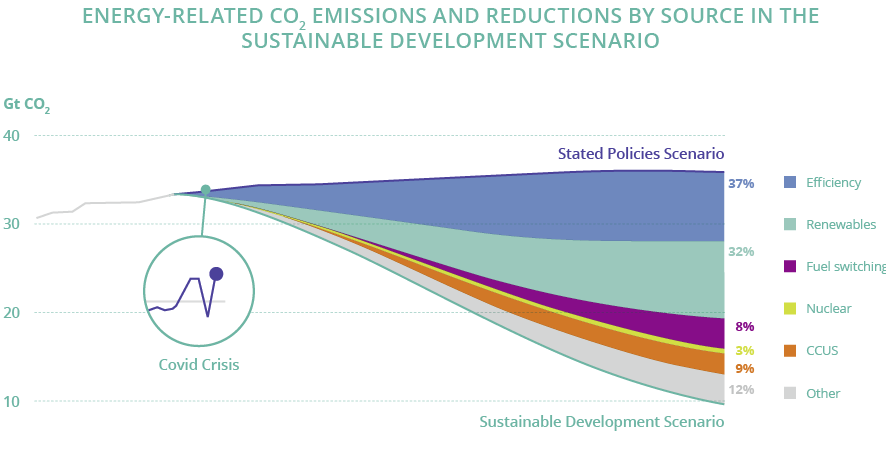
3.2.2.1 Application trends
At present, 75 percent of total greenhouse gas emissions in the EU come from the energy sector . The energy world is undergoing a radical transformation: promoted e.g. by EU and national roadmaps, the globally installed capacity of renewable generation has doubled within the past 10 years . Europe alone expanded its renewable generation capacity by 6.4% in 2021 . This increase is dominated by wind and solar energy being characterized by strongly intermittent, distributed generation. Altogether wind and solar energy made up one fifth of Europe’s electricity generation in 2021 with plant capacities ranging from domestic solar (≤ 10 kW) via commercial solar and wind (≤ 500 kW) to power stations at utility scale (≥ 1 MW) . At the same time, the levelized cost of electricity (LCOE) from photovoltaic (PV) sources dropped by 13 to 15%. However, the rise of renewables is still too slow - wind and solar generation growth must nearly triple to reach Europe’s 2030 green deal target. In the long term, it enables the substitution of fossil fuel-based transportation, domestic heating, and commercial & industrial processes as well as address the strong economic growth of non-OECD countries. Since the pursuit of all economically viable opportunities for efficiency improvement can reduce global energy intensity by more than 3% each year, increasing energy efficiency may be accountable for 30 percent CO2 emission-reduction by 2050 with current policy settings, but can be even increased up to 40 percent if worldwide announced climate pledges are met. Energy supply to all sectors affordably and reliably needs to match the demand and availability as efficient as possible (Figure F.54).
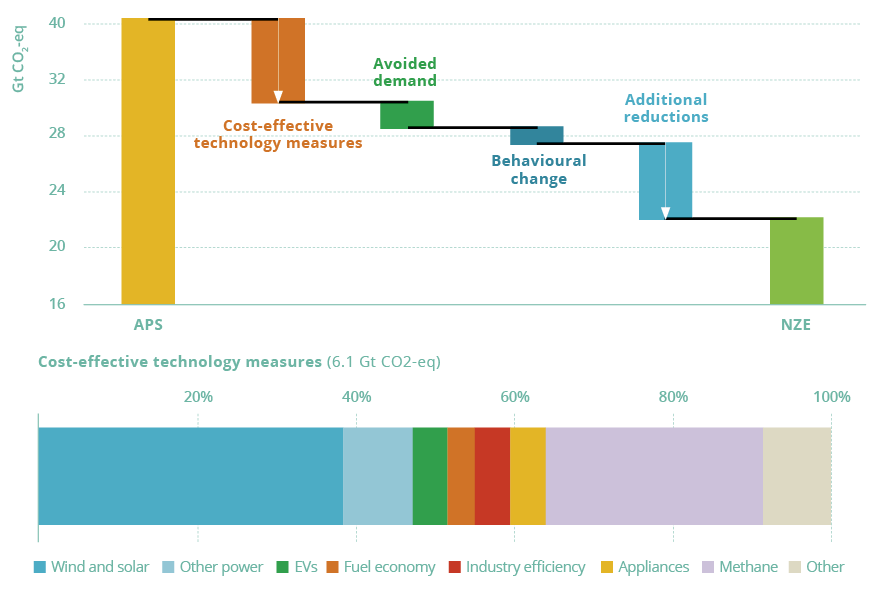
Thus, the power grid architecture developed for centralised, unidirectional, demand driven power generation will be transformed into a multi-modal energy system (MM-ENS) architecture (Figure F.55). It will comprise distributed renewable generation, energy conversion units for sector coupling, transmission and distribution grids allowing bi-directional power flow, and energy storage for all modes of energy (electric, thermal, chemical). Energy management systems (EMS) will optimize ENS-operation. It will match load and demand at all levels ranging from the nanogrid (behind the meter, building level) and the microgrid (district or community level) to the regional distribution grid, which is connected to the cross-regional transmission infrastructure. Fossil-fuelled power plants, which used to operate on schedules orienting at the demand, will turn into back-up power supply facilities.
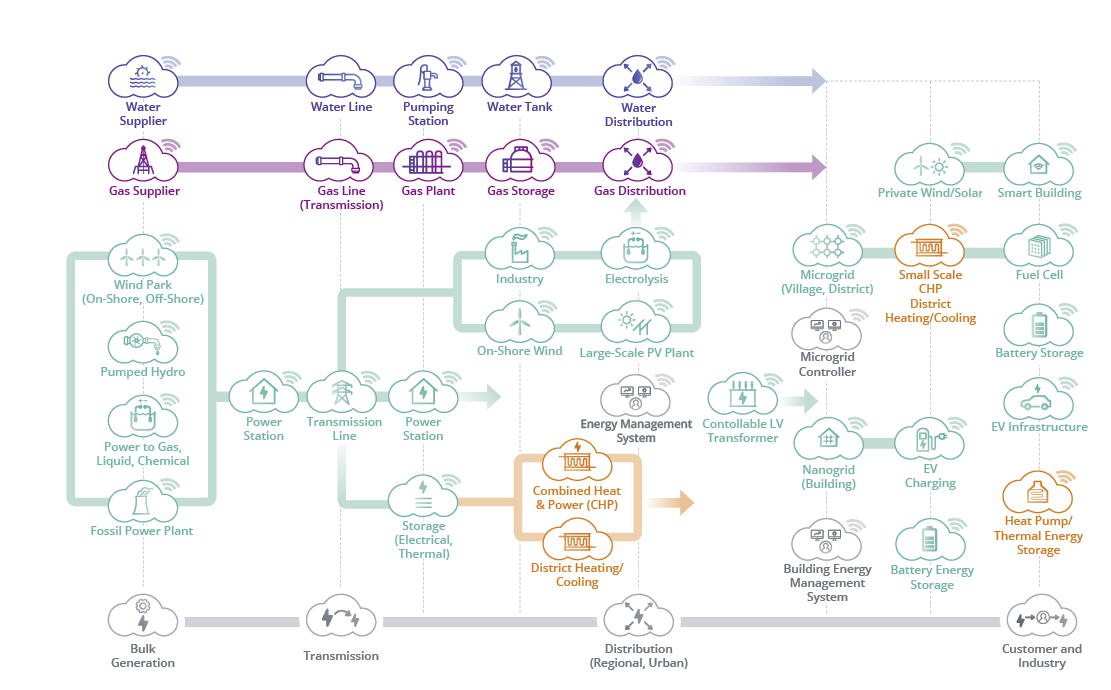
The overall reduction of energy consumption in addition to efficiency measures will be always a target, since all energy usage that can be avoided also implies reduction of emissions. This can be achieved by control elements for switching off energy use and zero power stand-by functionality or by transformation to new technologies as in the last decade the transfer to LED illumination had a high impact. Upcoming threats are energy consuming ICT technology related applications like blockchain, AI, data traffic, or digital currencies. The challenge will be to develop highly efficient algorithms and methodologies to decrease energy consumption despite the increased use of these new technologies.
Key to these new energy applications will be smart sensors, networks of sensors, and smart actuators that enable status monitoring on each grid level as well as smart converters (for all voltage levels). The converters need to use highly efficient and fast semiconductor power devices and modules that enable real-time control of energy system components and grids for optimized operation based on forecasts of generation and demand but also in case of any critical event. The future grid operation requires a sophisticated information and communication infrastructure including cloud services, IT security, and AI technologies. Altogether, they will contribute to significant reduction of energy consumption and, consequently, CO2 emission.
To achieve the targets of the Green Deal and to have competitive advantages for European based technologies and solutions, research has to be performed in the following areas:
01. Significant reduction and recovery of losses (application and SoA-related).
02. Increase of power density and reduction of losses (e.g. through exploitation of new materials) and a decrease of system size by miniaturisation and integration, on the system and power electronics level.
03. Increased functionality, reliability, and lifetime (incl. sensors & actuators, ECS HW/SW, semiconductor power devices, artificial intelligence, machine learning, monitoring systems, etc.).
04. Manufacturing and supply of energy relevant components, modules, and systems.
05. Management of renewables via intermediate storage, smart control systems, share of renewable energies, peak control or viability management for the increase of energy flexibility. Grid stabilization through e-vehicle charging.
06. Energy supply infrastructure for e-mobility, digital live, and industry 4.0.
07. “Plug and play integration” of ECS into self-organised grids and multi-modal systems, real- time digital twin capability in component and complete system design (to simulate system behaviour).
08. Safety and security issues of self-organised grids and multi-modal systems through smart edge devices and high level IT security (resilient communications and trustworthy AI).
09. ECS for energy storage technologies: production, transportation, storage, distribution, combustion and energy conversion systems.
10. Optimisation of applications and exploitation of achieved technology advances in all areas where electrical energy is consumed.
11. Energy technologies in the circular economy approach: predictive and condition-based maintenance with repair and recycle capabilities.
12. Aligning with standardization of our energy systems.
13. Manufacturing and world-leading technologies for energy relevant applications in Europe.
14. Scheduling for cost-efficient energy consumption.
15. Involvement of the consumer: traceable eco-footprint and incentives towards environmentally-friendly behavioural change.
3.2.2.2 External requirements and Societal Benefits
In alignment with the Parisian Agreements, the EU committed to substantial reductions of CO2 emission. In particular, the EU aims to make Europe the first climate-neutral continent by 2050 (EU long-term strategy) while boosting the competitiveness of the European industry. Carbon pricing throughout the EU economy is going to be implemented more strictly. Further climate laws will be introduced and continuing policies will be clarified by the European Commission in 2022. The new policy regarding “Clean energy for all Europeans package” was completed by the EU in 2019 as a comprehensive update of its energy policy framework and updated with the new Green Deal in July 2021. It emphasizes renewable energy, energy performance of buildings, energy efficiency, governance regulation, and electricity market design. Smarter buildings with more automation and control systems for effective operation shall be promoted. E-mobility infrastructure is going to be supported further. Energy efficiency targets and energy labels were tightened to encourage the industry to innovate.
To achieve the Green Deal goal of “clean, affordable and secure energy” in all sectors, new laws and regulations will be required. While subsidies and regulations will promote sustainable developments in all application domain of ECS (energy, industry, mobility, communication, consumer goods, and cities), the energy domain with targeted 40% renewables in the energy mix until 2030 is the foundation to all of them. Additional perspectives are given by the United Nation’s “Roadmap 2050” addressing sustainable development solutions and implementations towards a carbon-neutral global population.
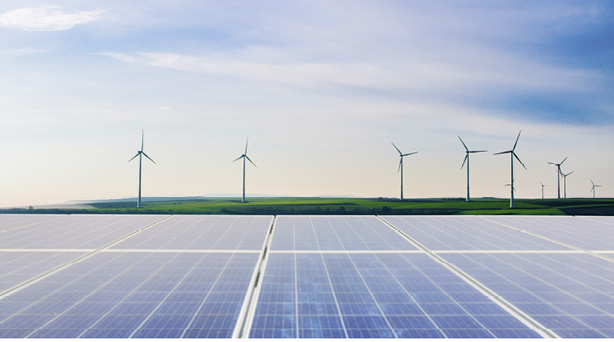
All these factors are considered for the roadmaps on research, development, and innovation of ECS for the applications in the energy sector. Potential targets comprise the implementation of electricity storage solutions (e.g. vehicle2grid, battery grid storage), the further increase in efficiency and the reduction in life cycle costs of energy generation from renewable sources (Figure F.56), the electrification of transportation (Figure F.57), and the thermal processes in industry as well as the development of secure, self-learning energy management systems for buildings and industrial sites. ECS as enablers support the EU and national energy targets to achieve sustainability (Figure F.58) and are essential for a highly developed energy landscape towards a fair, democratic, healthy and prosperous society.
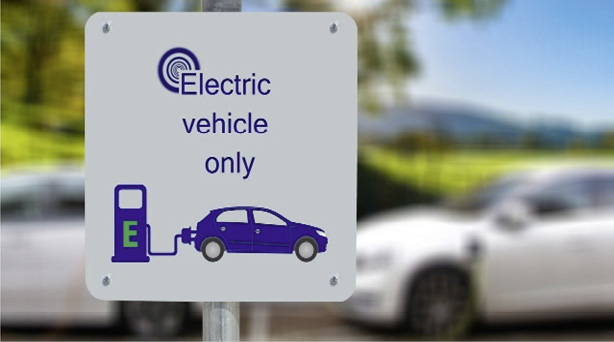
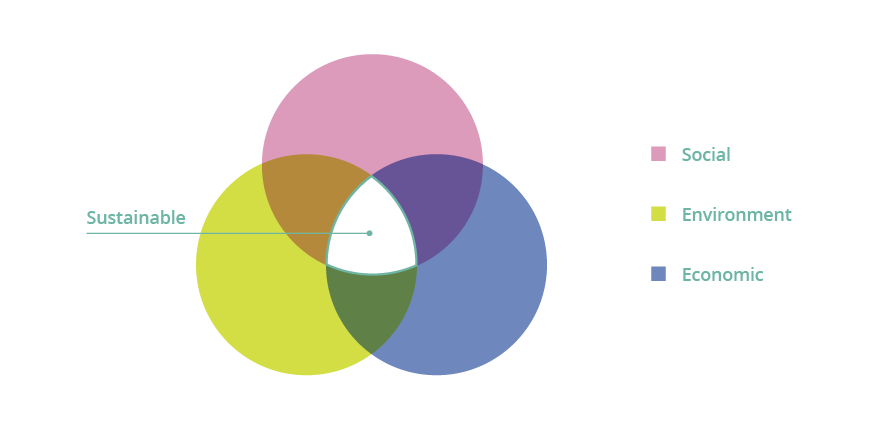
Energy efficiency through ECS fosters economic development towards a circular economy and new employment opportunities. They will have a huge impact on job generation and education if based on the complete supply chain and fully developed in Europe. With more than 11 million jobs in the field of renewable energies155 and indirectly involved technologies, this is a visible and significant factor for economic and societal stability. The capability of maintaining the understanding of the complete systems as well as the competence from small-scale solutions up to balanced regional energy supply solutions are key to the European competitiveness and success in the global market of energy solutions. Also the consumer itself can contribute its share, thus consumer empowerment to energy savings and efficiency should be taken into account for the development of energy systems.
Societal benefits include access to knowledge, development of modern lifestyle and the availability of energy all the time and everywhere - with a minimum of wasted energy and a minimum of greenhouse gas emissions. Therefore, ECS and its application domains enable Europe to meet the needs of the present without compromising the ability of future generations to meet their own needs.
European ECS companies are among the leaders in smart energy related markets, e.g. for electrical drives, grid technologies, and decentralised renewable energy sources. Four European-based power semiconductor suppliers are among the top 20 in the world, having a combined market share of over 22% in 2021. Two of them amongst the top 5 including the market leader156. The major growth driver there includes growth in renewable energy sectors such as solar power generation157. Three power modules suppliers are found among the top 10 with one worldwide leader in the automotive, in the discrete power device and in the security-IC sectors, respectively, while the combined market share is more than 35%. Overall, the share of European suppliers in this growing market is increasing, which underlines their competitiveness. The companies invest in Europe and expand fab capacity or even build up new semiconductor fabs in Europe. This position will be strengthened so that further employment is secured by innovative research within Europe. The technological progress will have a multiplying effect by creating a convergence between semiconductor and other promising future technologies like 6G, IoT, AI, and cloud-edge computing. As a result, EU ECS market prospects are seen very strong158. A seamless line from ECS R&D&I to production covering future energy businesses from generation over conversion to distribution and transmission ensures Europe’s technological non-dependence.
Since the beginning of the Covid19-crisis, the ECS industry moved to protect employees, and secured supply chains. Despite the crisis, the ECS industry recorded on average a double-digit growth in 2020, with the fourth quarter marking the best quarterly result to date. In contrast to other industries, which had to contend with declining demand, the ECS industry experienced the opposite development and consequently suffered supply bottlenecks for electronic components. In the future, the focus on a strong R&D strategy is essential to emerge stronger from the crisis. Targeted investments in innovation can create a long-term competitive advantage and fill knowledge gaps. Especially the investments in new technologies rather than simply focusing on product variation or optimization to extend the product life cycle leads to the achievement of a pioneering role and should therefore be pushed159. ECS enable affordable energy conversion efficiencies of 93% – 99%, which improve the use of renewable energy resources. Involving new materials such as wide band-gap semiconductors, new device architectures, innovative new circuit topologies, architectures, and algorithms, the total system cost can be lowered. The focus on ECS development secures a smooth implementation of renewable energy power plants into the EU grid, a step towards the long-term goal for 2050. To further ensure a competitive, self-sufficient and efficient energy transmission and consumption in the EU, the energy highway through Europe, decentralized intermittent energy sources, bi-directional grid and storage systems, and distributed AC/DC network and grid technologies need to be implemented. These measures will support the EU by reaching its goals of a connected, breakdown and blackout protected, market-based, and yet more consumer-oriented energy market. Consequently, EU’s energy system will serve as blueprint for global application. The scenery was already set in the recent years – with the change in the supply strategy to be independent of strategically critical gas and oil the conversion has to be faster and the change heat pumps and battery electric vehicles in combination with the Green Deal targets will even need a faster development for the conversion to a highly dynamic energy grid for the provision of renewable energies.
Five Major Challenges have been identified for the energy domain:
- Major Challenge 1: Smart & Efficient - Managing Energy Generation, Conversion, and Storage Systems.
- Major Challenge 2: Energy Management from On-Site to Distribution Systems.
- Major Challenge 3: Future Transmission Grids.
- Major Challenge 4: Achieving Clean, Efficient & Resilient Urban Regional Energy Supply.
- Major Challenge 5: Cross-Sectional Tasks for Energy System Monitoring & Control.
3.2.4.1 Major Challenge 1: Smart & Efficient - Managing Energy Generation, Conversion, and Storage Systems
According to the IEA’s Efficient World Strategy, digitalization enhances energy efficiency gains in the transportation and industry sectors160. Smart and efficient energy systems are drivers of energy savings. Therefore, they are in full alignment with the Green Deal. Alternative ways of energy generation (hydro, photovoltaic, and wind) and the electrification within the industry, the transport / mobility, and the construction / building sectors result in the challenge of creating smart, efficient, and reliable energy generation, conversion, and storage components.
Smart Energy Systems
For operating smart energy systems, all the energy conversion and storage components need to be equipped with smart actuators and sensors for status and health monitoring as well as optimization of grid operation. The integration of sensor, connectivity and edge processing in supplementary/additional parts will enable the creation of intelligent facilities by retrofitting. The creation of secure electronic control units requires development of specific hardware and software.
Consequently, smart control units need to be developed for all types of energy production, conversion, and storage components comprising smart electronic converters, actuators, sensors, security systems and reference communication interfaces. They shall have plug-and-play functionality and real-time digital twin capabilities in component and complete system design to simulate system behavior for evaluation of its’ health status.
For offshore energy generation, such as windfarms and tidal energy generators, fibre optical sensors is an emerging technology beneficial for online monitoring of metal fatigue and excessive turbulences. This technology is currently being developed for such monitoring in aircraft wings and ship masts.
Conversion
Electrification of industry is one of the main implications to reach the 2050 decarbonization targets, mainly via the conversion from fuel-based heating processes to electro-heating solutions. In addition, direct electrification of industrial production processes (such as electro-synthesis of chemicals or electrolysis) is also crucial for replacing present CO2 emitting solutions161. In the case of Heating, Ventilation, and Air Conditioning (HVAC) systems, significant reductions in consumption can be obtained by optimizing the system that handles all the processes of energy management or by changing the use of the Machine-to-Machine (M2M) technologies. For both strategies, efficient ECS are required to obtain optimal control functionality based on sensing, collecting, processing, and evaluating device related data162. DC power supply requirements based on advanced semiconductor power devices will provide lower power consumption and thus, feature higher efficiency of the increasing ICT energy consumption (i.e. through data centres) 163. Investments in the next-generation computing, storage, and heat removal technologies will be required to avoid a steep increase on energy demands and to minimize the implications of unavoidable data centre energy use on the global climate164. In data centres and 5G/6G networks, photonic ICs can route information streams from fibre to fibre without conversions into electronics in between. This will be highly efficient and save energy.The advanced features of 5G/6G will innovate the use of the technology (Figure F.59), but as consequence of larger data rates and through-puts, cost and energy demand will increase substantially. Therefore, energy harvesting capability of sensors and devices in the 6G environment will be one of the crucial aspects towards a green and cost-efficient technology landscape165.
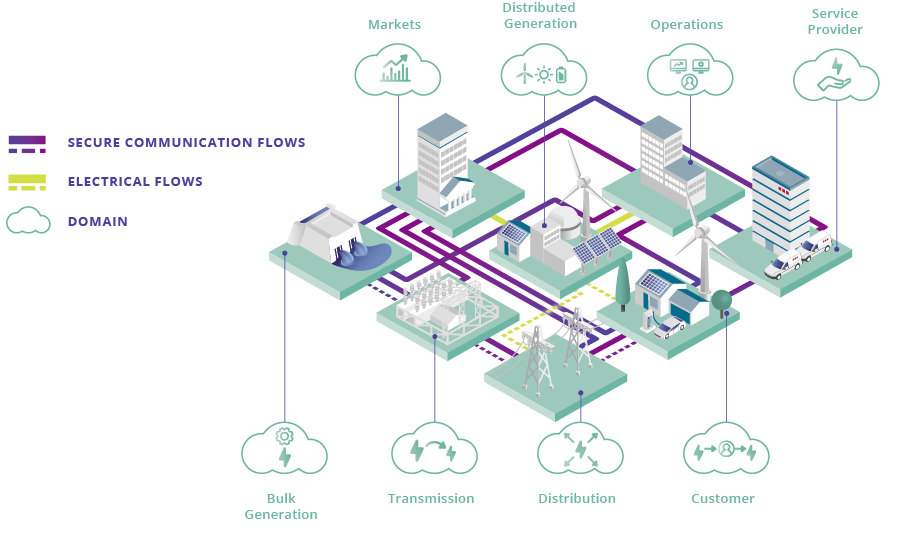
Power electronics circuits based on semiconductor power devices are used in all conversion processes. Silicon based power devices are approaching their ultimate limits in terms of breakdown voltage, current, switching frequency and temperature capabilities. Next generation power semiconductor devices will rely on Wide Band Gap (SiC, GaN) and Ultra WBG (diamond, Ga2O3) technologies. Due to this unstoppable trend, research on device reliability, packaging and assembling methods suitable for very high electric fields and high temperature, is strongly required. A focus also needs to be set on the medium voltage grid (< 45 kV).
Storage
Energy storage deployment provides energy system flexibility166. Looking at further storage possibilities, different options for various capabilities need further efficiency improvements. As an example, optimized converters, sensor solutions for monitoring, and battery management systems need to be developed for storage options, all including ECS. In power generation, hydrogen with its many uses (Figure F.60) is one of the leading options for storing renewable energy. Hydrogen can be used in gas turbines to increase power system flexibility. In combination with fuel cells, it is also a great vector of clean energy since it allows to produce electricity directly onboard of EV or in areas, which are cut off from the power grid. With declining costs for renewable electricity, interest is growing in electrolytic hydrogen167. ECS will be employed in electronics for electrolyzers, fuel cells, as well as power management and health monitoring.
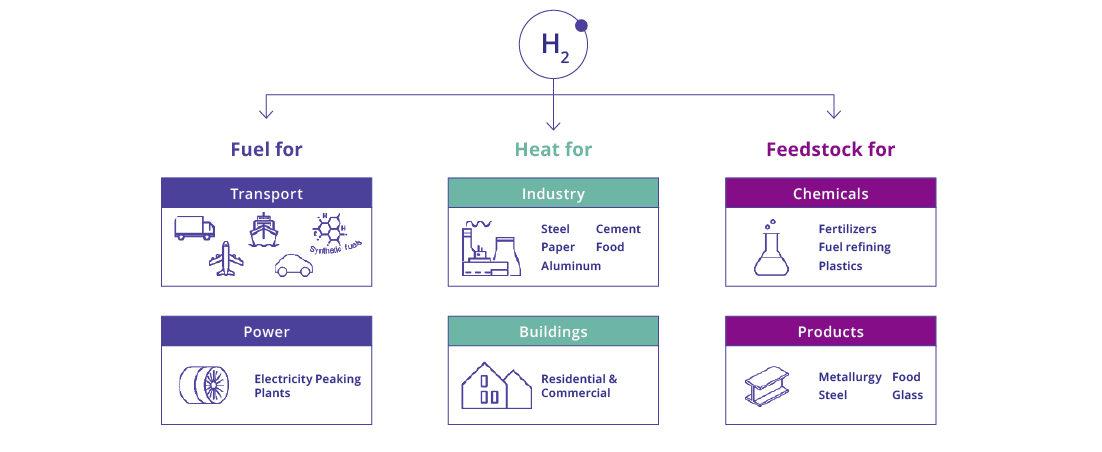
- Increased efficiency at all levels:
- Residential, commercial, and industrial demand side management (scheduling and load adaption):
- Sensors, actuators, drives, controls and innovative components.
- Full monitoring in adaptive and controlled systems.
- High efficiency electric drives, heat pumps, cooling, HVAC, data centres and other consumers of electricity for variable load operation.
- Solutions for increasing power demand of 5G/6G systems.
- Development of Energy Management Systems including:
- Optimization module.
- Demand and generation forecast.
- Customer preferences.
- Weather forecasts.
- Price/tariff information/forecast for scheduling controllable loads and generators.
- Smart sensor network: internal and external physical parameters that influence energy conversion efficiency.
- Resilient and smart communication and edge devices.
- Deployment of Trustworthy AI.
- Fiberoptic sensors for fatigue detection.
- Converters for power quality improvement (e.g. electronics filters to manage resonances).
- Sensors and controls for the management of decompression and compression and leakage detectors for methane, hydrogen, and other gases.
- ECS for the coupling of processes in the chemical and electrical industry.
- Traceability and labelling of green energy.
- Conversion of industrial processes:
- “Industrial electrification” (Replacement of CO2-emitting processes by others based on “clean” electricity).
- Electric drives for commercial & industry applications.
- Industry 4.0 with combination of Cyber-Physical Systems (CPS), Internet of Things (IoT), Artificial Intelligence (AI).
- DC subsystems for industrial production / data centre applications and DC distribution grids.
- Photonic routing in data centres from fibre to fibre without conversion to electronics.
- Carbon capture technologies compensating production emissions (up to negative emissions).
- Development and application of storage optimized for residential, commercial, industrial utilization:
- Control, interfaces to batteries, fuel cells, hydrogen storage electrolysers.
- Integrated battery driven applications (e-car charging, PV – system local storage).
- Power Storage to "buffer" net fluctuations and to avoid long distance transmission.
- Smart storage technologies from low to medium voltage.
3.2.4.2 Major Challenge 2: Energy Management from On-Site to Distribution Systems
The distribution grid comprises commercial scale renewable generation as well as private smaller renewable power generation units, conversion between different energy modes, storage, control and protection systems for the grid infrastructure together with all kind of consumption.
Autonomous Control Systems
In the future distribution grid, generation and consumption by power electronics systems will surpass the share of synchronous generation. This leads to potential grid instabilities due to lack of inertia. Therefore, autonomous control systems need to be implemented to control the high demand loads. These control systems should be organized hierarchically to adjust the heavy loads according to the actual local production and storage capabilities so that import or export of power is minimized. Price control systems such as TOU (Time of use) can help to prevent grid violations. Storage devices, such as local community storages or e-Vehicles, can be charged when the price is low and discharged when the price is high, to provide flexibility as well as to ensure stability and reliability in the grids.
For industry or larger groups of buildings, control methods increase the flexibility of the total system and can be set up using hierarchical and intelligent control methods to minimize costs and to provide peak-shaving (Figure F.61). For larger power production facilities, hybrid generation and storage solutions are also discussed, which integrate the power production facilities with storage devices to have the best arbitrage cost. Novel grid architectures for manufacturing strive to increase topological and energy flexibility within production cells to enable adaptive production optimization. Also, blackouts and its’ consequences need to be prevented, since for example for large industrial electrolyzers they result in serious safety and cost issues.
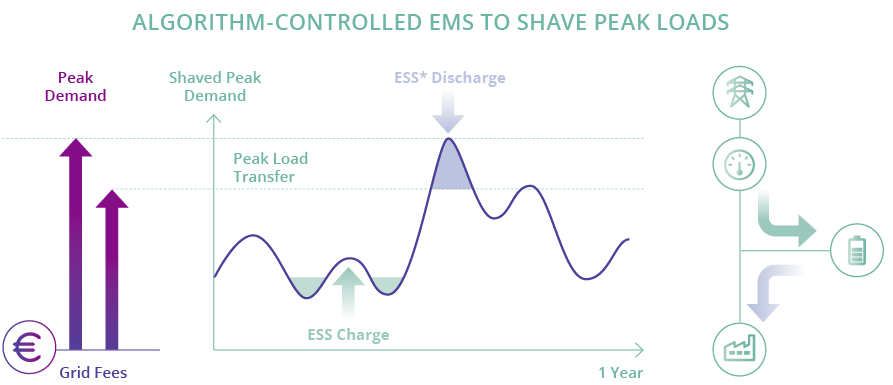
Security, Reliability and Stability of Energy Systems
For stable, resilient on-site energy systems, multi-modal energy management systems allowing integration of electricity, heating & cooling, molecules, and transport (e-vehicle charging incl. Vehicle-2-Grid) will be developed. Their features comprise high level IT security, energy trading via local energy market platforms, renewable energy certification, development of solutions for low voltage electronic systems that are easy to setup, as well as support for self-learning against evolving needs.
Energy Management Systems for industrial and residential customers include optimization module, demand and generation forecast, customer preferences, weather forecasts and price/tariff information/forecast. They require beyond-state-of-the-art techniques for scheduling controllable loads and generators, and to forecast the weather to produce accurate generation profiles. Furthermore, the interface to the grid might be used for additional power quality services based on power electronics converter technologies beyond state-of-the-art reactive power compensation (e.g. virtual inertia and balancing).
- Security, reliability and stability of total energy system:
- Automation of grids.
- Storage of data.
- Trustful AI and ML for optimized operation of the grid.
- Machine-learning based forecasting algorithms for generating accurate generation profiles of expected power production and consumption.
- Deployment of sensors and edge computing devices to health-check grid assets to increase lifetime and optimize operation.
- Converters for power quality improvement (e.g. electronics filters to manage resonances).
- Stable and Resilient On-Site Energy:
- Integration of electricity, heating & cooling, molecules, and transport (e.g. Vehicle2X).
- Coupling with energy trading systems, e.g. local energy market platforms.
- High level IT security.
- Renewable energy certification.
- Hybrid solutions:
- Integrating power production facilities with storage devices.
- Arbitrage cost, keeping level of production according to market bid.
- Virtual markets:
- Flexibility in demand & supply.
- Aggregation of Energy consumption and production.
- Electric energy supply for manufacturing:
- Plug-and-play capability for components, self-learning:
- Integration of low voltage systems using flexible planning rules.
- Cost effective solutions to minimize set up-time and manual parametrization.
- Reduced physical size and weight of individual transformer stations with equivalent power ratings.
- Development of solid-state transformers with:
- New functions for the operation of power systems.
- Avoidance of infrastructure extensions caused by increasing share of distributed generation.
3.2.4.3 Major Challenge 3: Future Transmission Grids
New grid challenges
Future transmission and distribution grids will remain an integral backbone of energy systems. Coupling of different domains like electricity, thermal, gas etc. will enable new business opportunities which require new technological solutions for high power electronics, combined with sensors and ICT for monitoring, intensive control and prediction.
The energy generation and energy consumption pattern will drastically change as the industry and society at large will be highly electrified. Base industries such as the chemical industry, steel and cement production will completely change production technology to enable fossil free production and will require extreme amounts of electric energy. New industries such as giga volume battery production factories are planned in several places in Europe168. This together with a massive expansion of supercharger station for private passenger cars and heavy trucks with individual charge capacity of more than 1 MW will put severe challenges on the grid capacity in both networking and electronic components to manage highest possible efficiency. Therefore, further ECS R&D needs to work towards improvements of the grid capacity with the highest possible efficiency (Figure F.62). Thus, continued development of components for HV transmission for 1.2 MV or even higher voltages are needed to roll out an efficient energy transmission over Europe. In addition, new business models must be developed for the electric energy market enabled by the smart grid technology.
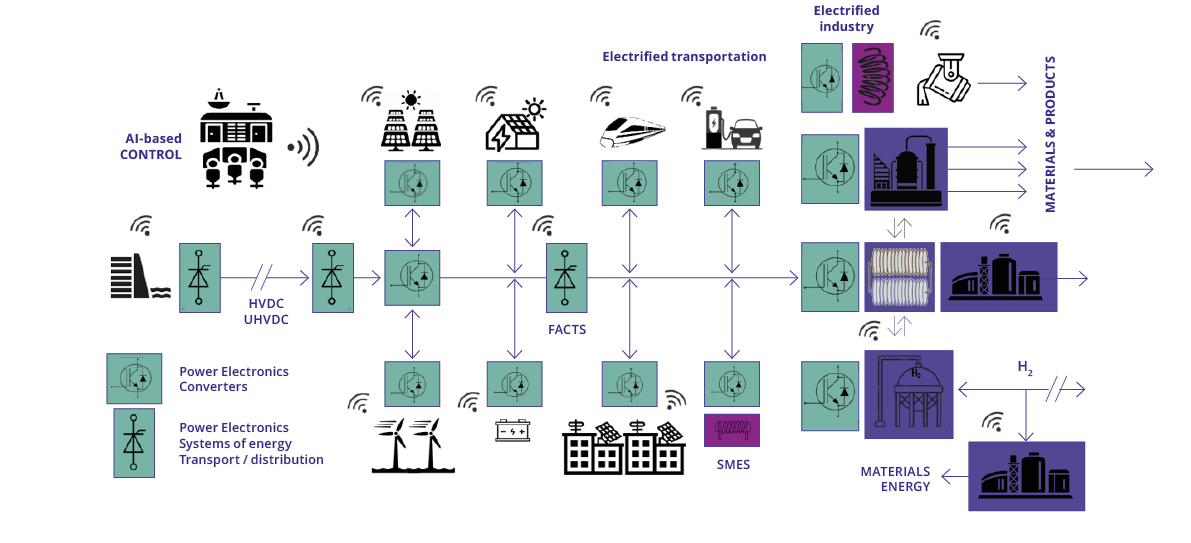
Resilience
To account for adverse conditions caused by climate change, the new national and transnational grids must include autonomous electricity generators based on fuel cells or local storage systems for communications and network information management as well as water-resistant components or modules. Also, sensors need to be placed at critical points to immediately alert authorities in case of unexpected incidents. To be able to quickly react to an electricity line fault, the system will benefit from powerful switches and AI to successfully reroute the systems. Additionally, predictive maintenance (e.g. with digital twins) of the energy supply sensors provides further safety and resilience. Due to the weak tectonic movement in most of the parts in Europe, transmission grids could become much more resilient and loss-less when buried in the ground. Thus, extra isolation technology needs to be considered and critical points equipped with smart ECS for monitoring, control, and prediction.
- Grid stability during the industrial transition:
- Efficiency increases.
- Development of smart medium voltage grid.
- Development of components for HV transmission for 1-2 MV or even higher voltages.
- New solutions for high power electronics, combined with sensors and ICT for monitoring, control and prediction.
- Development of new simulation and business models to foster innovations regarding grid stability.
- Development of a Trans-European energy infrastructure:
- Secure, cross-regional transmission infrastructure.
- Multi-terminal HVDC systems connecting remote energy generation sites.
- Interaction between distribution systems on community and district level.
- Development of components for HV transmission for > 1.2 MV.
- Minimize Losses.
- Requirements on ECS by disruptive changes in transmission and use:
- Flexibility in system design and operation.
- Water-resistant components/modules.
- Autonomous electricity generators based on fuel cells.
- Modelling, sensing and forecasting weather conditions and thus, supply and demand.
- Intelligent power devices, systems, and switches.
- Status-/health-monitoring (e.g. ice sensor/detection) for transmission lines.
- ECS for multi-modal energy systems.
3.2.4.4 Major Challenge 4: Achieving Clean, Efficient & Resilient Urban/ Regional Energy Supply
A 40% renewable energy share in the electricity sector in Europe by 2030 needs additional decentralized, intermittent energy sources, bi-directional grid and storage for energy supply in transport, industrial and smart cities applications.
Multi-energy Systems (MES)
MES help to achieve optimized energy management. All sectors are integrated to maximize overall system efficiency. Energy flows between sectors and their storages ensure the highest use of renewable energy while balancing fluctuations.
Heating supply uses district heating, supported by heat-pumps and boilers, using thermal storage in the district heating system (Figure F.63). Integration with industry makes use of waste process energy using heat pumps to boost from low (40-50 deg) to high temperatures in the pipe (80-90 deg). Electrolyzers add to the gas system or transport. Water treatment uses excess power from renewables adding further flexibility.
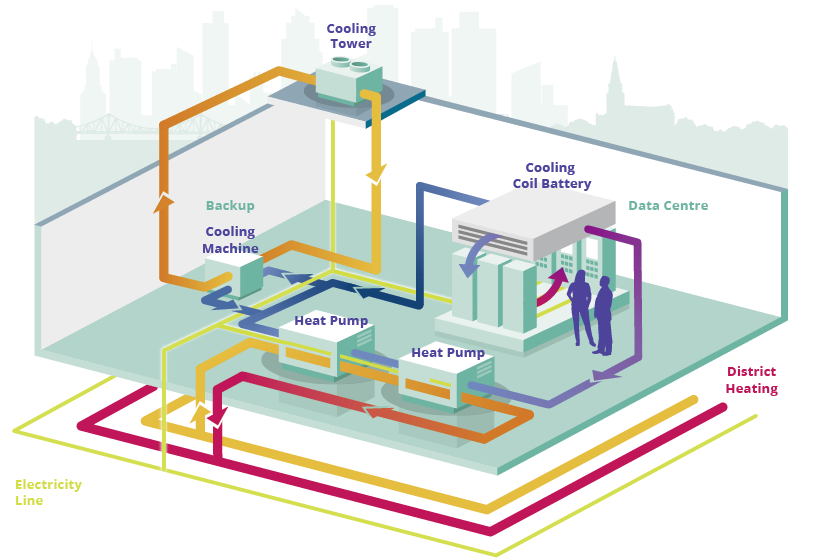
Local communities use MES concepts on regional level. Different local inputs are gathered for an overall aggregated control for the larger regions as well. Autonomous controllers are used behind the meters to support overall control. A clear hierarchical set up, control structure and knowledge of market interactions are necessary.
Complex integrated control systems use AI, machine learning and comprehensive communication grid/IoT platforms (including edge computing) to get all data for control and optimization. Risk and security analysis provide resilience and ensure stability of MES.
Urban Transformation
Emission free cities use electrification and decentralised storages to improve efficiency and reliability. ECS as indispensable components ensure efficient management of data and data storage. AI approaches and the ECS supply chain for integrated applications in energy are key enablers for smart power grids. Electrification of urban mobility supports individual and public transport (incl. utility EV) and furthermore, contributes to the stabilization of the grid. The first needs household and public charging, the latter uses well defined charging points on (bus) lines or at terminals. Powers vary from 10 kW (LV) to 600 kW (MV). Reservation and optimization are based on ICT.
Other, crucial aspects of emission free cities are an efficient urban energy infrastructure, low carbon and smart residential and service buildings, low carbon mobility, smart water systems and smart waste management. Even the shift to LEDs without any smart functions can result in energy savings of ~50% in an industrial setting169. Carbon capture technologies will add another dimension to the energy systems.
Storage Solutions
In households, battery energy storage devices can used to increase self-consumption. Some regions will use heat/cooling storage. Algorithms/models for optimal use of storage (community/private/ industrial) are based on technical parameters, demand and generation forecasts, customer preferences, in order to reduce power peaks and to support integration of RES into existing infrastructure.
MES in larger communities with different kinds of storage possibilities (electrical, thermal, gas, water etc.) play an important role. V2X is used as huge distributed electrical energy storage. Systems with electrolyzers might use storage tanks for gas production. Thus, development of grid-supporting control algorithms and supporting regional energy management for communities (e.g. P2P trading via storage systems, self-consumption optimization) are needed.
- Electric Energy Supply for urban mobility:
- Development of household and public charging infrastructure.
- Creation of HV (wireless) charging points along the (bus) line or at fleet terminals, for public transport.
- Reservation and optimization services implemented with ICT solutions.
- Electric Energy Supply for urban life:
- Increase share of renewable generation, self-consumption (mainly heating/cooling and EV) and building optimization.
- Local DC-coupling of various technologies for fast charging at home.
- Regional Energy Distribution infrastructure:
- communication infrastructure to support self-organized local energy communities.
- sustainable off-grid supply with power electronics-based grid forming capabilities.
- Virtual power plant functionality optimizing match between generation and demand.
- Operation of connected energy systems:
- Storage systems:
- Development of grid-supporting and peak-shaving control algorithms.
- Support for regional energy management for communities.
- Peer-to-peer trading by using storage systems.
- Self-powering systems for small IoT nodes.
- Local energy harvesting to substitute battery powered devices and eliminate the high demand of energy for the battery manufacturing and distribution logistics.
3.2.4.5 Major Challenge 5: Cross-Sectional Tasks for Energy System Monitoring & Control
Focusing on current energy management platforms, they still have shortcomings in terms of automation, interaction and intelligence. Thus, when the traditional energy grid is evolving into a smart grid, it needs to integrate ICT and power electronics massively. The ECS empower the electrical utilities providers and consumers, improve efficiency and availability while constantly monitoring, controlling, and managing the demands. The huge complex networks need cross-sectional approaches for monitoring and control to achieve efficiency, security and reliability of the communication and electrical flows (Figure F.64) - all based on new ECS technologies.
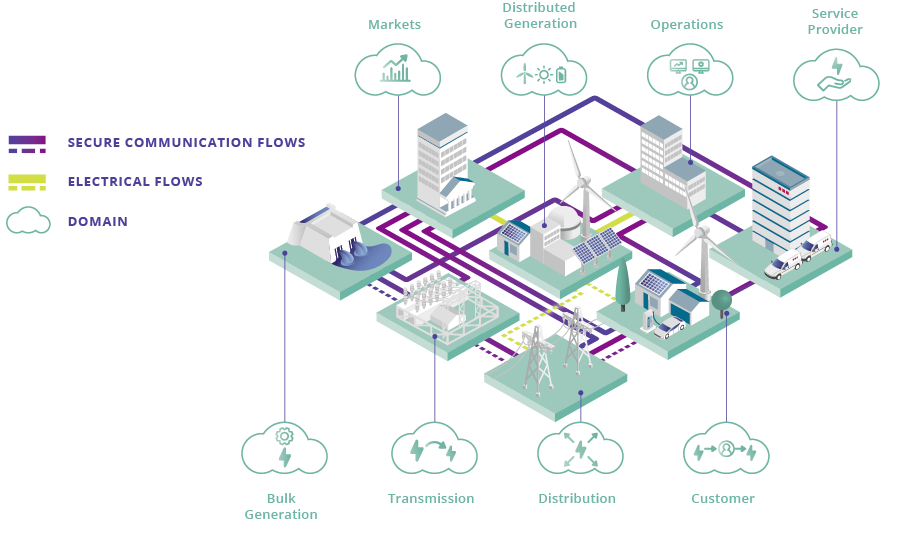
Optimization in Monitoring and Control
To ensure security, reliability and stability of the total energy system, it is important to know the current state of the system at all times. Therefore, observability and state estimation together with forecast of expected production and consumption play an important role. This requires automation of the grids, use of sensors at different levels, storage of data, AI and machine learning to operate the grids in an optimized way and at the same time obeying data security and GPDR. Data collection within the grid needs to be limited on chosen parameters to avoid unnecessary costs and complexity.
The IoT technology as application in the smart power grid can help to achieve sustainable energy, low latency, and reliability170.
Machine-learning used for forecasting energy demand in smart grid environment contributes to medium-term and long-term prediction of consumption and production and is able to solve energy management issues through improved accuracy of prediction171. It allows administrators to optimize and plan their resources and manage energy inconstancies and variations.
Nevertheless, security concerns and vulnerabilities need to be identified in today’s electricity grid and sufficient solutions implemented to reduce the risks to an acceptable secure level172.
Energy Management Platforms for integrated energy systems
The European electrical power system is undertaking a transformation process driven by targets towards renewable energy sources. A challenge will be that all different energy infrastructures (electric, thermal, molecules) will be interconnected on high, mid and low power/voltage scale but all have completely different time scales of response. Different energy sources from different operators can be managed through ML, algorithmic trading, agile transformation, etc. In this way, challenges of current and future applications like the energy transition and the digital revolution can be faced appropriately173. Energy Management Systems (EMS) are required to enable efficient and combined operation of multiple energy systems and components. Within a study that quantitatively examined 98 scientific papers dedicated to EMS in buildings and households, the identified focus areas were mostly the reduction of energy costs or peaks, as well as the increase of comfort. Results show that high computation time is a significant weakness of current EMS. A possible solution to that could be heuristic algorithms. Furthermore, the study suggests that stronger focus on high uncertainties and robustness is needed in order to transfer EMS with operational management and scheduling into practice. The integration of forecast methods also needs further attention. Regarding sector coupling (e.g. heat and electricity), major challenges exist due to great complexity and uncertainties over longer optimization horizons. Moreover, multi-level EMS in combination with cloud computing offer exciting approaches for new research questions174.
Hardware
Electrical grids aim to become more distributed, smart, and flexible to meet the increasing electricity demand. For new grids, the trend is to design energy generation and consumption areas together, in distributed form. Therefore, especially power electronic devices play a crucial role to regulate distributed generation and dispersed energy-storage devices together and into the grid. Future power converters also act as edge devices actively contributing to a stable grid either in grid forming devices, virtual inertia and other functions. Hence, the intensive use of power electronic converters in the microgrid brings their control methods to the forefront, which should meet good dynamic response and high reference tracking characteristics175. The domain of combining low power and high-power components does require fundamentally new HW solutions. It necessitates heterogeneous integration at the highest and most diverse levels, which leads to unprecedented EMC and thermo-mechanical concerns. It may open the door to developments possible in no other application field. Exemplary, while sensors (e.g. for self-monitoring) placed directly into power switches controlling the energy flow to an entire city, two heterogeneous worlds meet (e.g. kV and pW, MA and nA). The sensors must be able to withstand strong magnetic field changes and temperature fluctuations (300 degrees +), thus requiring research and innovation.
- Self-adaptive control based on Artificial Intelligence / Machine Learning:
- Data driven analytics (descriptive, diagnostic, predictive, and prescriptive) in smart grid.
- Fraud detection.
- Design, development, and application of deep learning in smart grid.
- Artificial intelligence in advanced metering infrastructure.
- Predictive and condition-based maintenance concepts resulting in reduced maintenance costs and increased lifetime for equipment and infrastructure.
- Algorithms for status, prediction & demand:
- Multiobjective optimization algorithms in smart grid; e.g. forecasting of generation and consumption.
- state-estimation based on measurement values, simulation values, trained models (machine learning).
- optimal utilization of storage systems (community storage, private storage, industrial storage systems) based on technical parameters, demand and generation forecasts, customer preferences.
- Short/long-term demand and generation forecast algorithms for different energy domains (electricity, warm water consumption, etc.) and integration into overall systems.
- New theories and applications of machine learning algorithms in smart grid.
- Data management, weather forecast, energy use forecast with a time horizon of 24 hours and with resolutions of at least 15 minutes (prevalent use of renewable solar, wind, hydroelectric sources according to demand profiles and use cases).
- Flexibility in management of energy supply and price offers to control the demand and avoid grid congestion:
Energy supply and energy efficiency are fundamental for all other applications from mobility and industry to societal sectors. The requirements on the ECS for the energy applications strongly spark the technology developments in (high-) power electronics but also for sensors, photonics, signal processing, and communication electronics along the full supply chain, i.e., from design to processing to integration. Hence, the “Energy” Chapter has close links to the "Embedded Software and Beyond", to the "Process Technological Equipment and Manufacturing" and to the "Components, Module, and System Integration" Chapters. Energy applications have specific and often particularly high requirements in terms of reliability, safety, and security, so that they are instrumental for the definition of the research work in the transversal Chapter on "Quality, Reliability, Safety and (Cyber-) Security". Also, the new communication infrastructure with a huge amount of new application results in an increased energy demand, giving rise to new challenges for the sustainable energy supply.
Due to recent progress in automotive propulsion concepts based on batteries, fuel cells, and hybrid engines, the synergies to the “Mobility” Chapter are particularly high. The new technologies requested for those applications require higher efficiency and reliability, which shows a strong connection to the high priority R&D&I areas listed in Chapter 3.2 “Energy”. The strongest interface is seen in the subjects of charging and storage infrastructure.
Finally, the Power Supply scenario with the availability and integration of several renewable sources with variable power generation profile, can be considered as an example of “System of Systems” (SoS), enabling the synergy with the related transversal SoS Chapter. Power management is fundamental for modern and future factories driven by Industry 4.0 concept, where digitalization plays a key role. Industrial IoT (IIoT), big data, artificial intelligence (AI) are enabling factors for energy-aware systems with full exploitation potential of synergy across several Chapters. Furthermore, a synergy with the “Connectivity” Chapter is fruitful to discuss future energy needs for 5G and 6G infrastructures.
153 European Environment Agency (2022). Share of energy consumption from renewable sources in Europe. https://www.eea.europa.eu/ims/share-of-energy-consumption-from
154 International Energy Agency (2021). World Energy Outlook 2021.
155 IRENA (2019), Renewable Energy and Jobs – Annual Review.
156 Omdia (2021), Power Semiconductor Market Share Database.
157 Market Research Future (2022). Power Semiconductor Market. https://www.marketresearchfuture.com/reports/power-semiconductor-market-1178
158 KPMG (2020), GSA Global Semiconductor Industry Outlook 2020 Part 2
159 McKinsey (2020). How the semiconductor industry can emerge stronger after the COVID-19 crisis. https://www.mckinsey.com/industries/advanced-electronics/our-insights/how-the-semiconductor-industry-can-emerge-stronger-after-the-covid-19-crisis
160 IEA (2019), Energy Efficiency.
161 EU (2018), European Commission, In-depth analysis in support of the commission communication, A clean planet for all: 773.
162 Chaouch et al. (2019), Energy Management in Smart Buildings by Using M2M Communication, IEEE Xplore.
163 EU (2016), Trends in Data Centre Energy Consumption under the European Code of Conduct for Data Centre Energy Efficiency. Joint Research Centre JRC, Directorate Energy, Climate and Transport.
164 Masanet et al. (2020), Recalibrating Global Data Center Energy-Use Estimates, Science 367, No. 6481: 984-986.
165 Routray (2016), Green initiatives in 5G, 2nd International Conference on Advances in Electrical, Electronics, Information, Communication and Bio-Informatics (AEEICB), Chennai: 617-621.
166 IEA Tracking Energy Integration, 2019.
167 IEA The Future of Hydrogen, 2019. https://www.iea.org/reports/the-future-of-hydrogen
168 Maisch, M. (2020). Europe’s Gigafactory Boom in Full Swing with Another Plant Announcement. PV Magazine.
169 Muneeb A, Ijaz S, Khalid S, Mughal A (2017) Research Study on Gained Energy Efficiency in a Commercial Setup by Replacing Conventional Lights with Modern Energy Saving Lights. J Archit Eng Tech 6: 202.
170 Jaradat, M., Jarrah, M., Bousselham, A., Jararweh, Y., & Al-Ayyoub, M. (2015). The Internet of Energy: Smart Sensor Networks and Big Data Management for Smart Grid, https://hdl.handle.net/10356/81241
171 Ahmad, Chen 2018: Potential of three variant machine-learning models for forecasting district level medium-term and long-term energy demand in smart grid environment. https://www.sciencedirect.com/science/article/abs/pii/S0360544218313811
172 Aloul, Al-Ali, Al-Dalky,Al-Mardini,El-Hajj 2012: Smart grid security: Threats, Vulnerabilities and Solutions.
173 Camponesci et al. (2020). ENEL Energy Management Evolution in a growing complexity of the Italian market context. https://ieeexplore.ieee.org/document/9241132
174 Schminke (2021). Overview of the current state of research on characteristics and algorithms of energy management systems in households and buildings. https://doi.org/10.1002/er.6738
175 Bayhan, Abu-Rub (2020). Smart Energy Mangement System for Distributed Generations in AC Microgrid. Quatar Environment and Energy Research Institute, Hamad Bin Khalifa University; Texas A&M University at Qatar, Doha, Qatar.