3 ECS Key Application Areas
3.4
Health & Wellbeing
Technological effervescence
The starting point of this Chapter is a simple statement (dating from 2006…) we have borrowed to the Harvard Business Review: “Why innovation in healthcare is so hard?”. Thousands of digital health solutions are on the market and thousands more are being developed, mostly by tech companies (including start-ups) entering the sector for the first time rather than traditional healthcare companies.
Many of these companies have great expectations, but they are learning that these expectations can be hard to realise in such a complex sector, particularly when it comes to securing payment for a solution.
Another issue lies in the conventional practices regarding the manufacturing of medical devices, considering that medical devices are developed in this context as a dedicated instrument for a specific application, limiting the expected return-on-investment.
However, gradually, change is coming. The objective of the Chapter is to highlight the business opportunity that the current acceleration of Health Digital Transformation should bring for the ECS community.
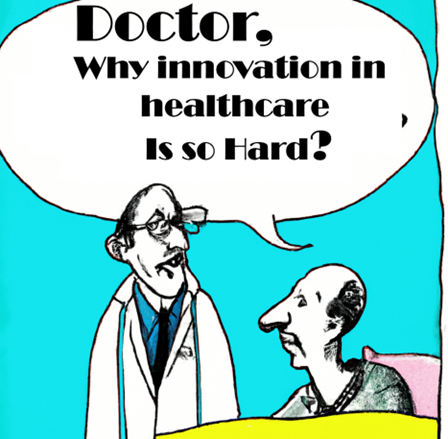
Healthcare systems sustainability
Current megatrends highlight the ubiquity of healthcare in our society. Urbanisation is directly correlated to population density with healthcare associated issues. The ageing demographic means more health issues like chronic diseases. The COVID 19 pandemics has disrupted access to health services at global level, while it already needed to be improved for several categories of the world population.
Healthcare costs are escalating worldwide. In 2040, the world will be collectively spending an estimated $20 trillion every year on healthcare, representing a 150% increase compared with 2014, according to a study published in The Lancet. This explains why almost all countries have established an explicit national Digital Health strategy associated with a roadmap to deploy solutions. Technology adoption, through the healthcare digital transformation, is considered as a pivotal element to secure the sustainability of health systems worldwide.
Opportunity for the European Electronic & Components System industry
The emergence of so-called P4 healthcare – predictive, preventive, personalised, participatory – as opposed to reactive healthcare, is blurring the lines between medical technology (MedTech), the pharmaceutical industry and electronic components and systems (ECS) industry, opening the way for healthcare innovation. This is a huge opportunity for the European ECS industry, its worldwide MedTech and pharma market leaders, as well as the 25,000 SME MedTech companies across Europe, since the new healthcare ecosystem will rely on digital instruments, advanced electronic sensors and photonics, microelectromechanical systems (MEMS), and the large volume, high-quality, low-cost production capabilities of the ECS industry.
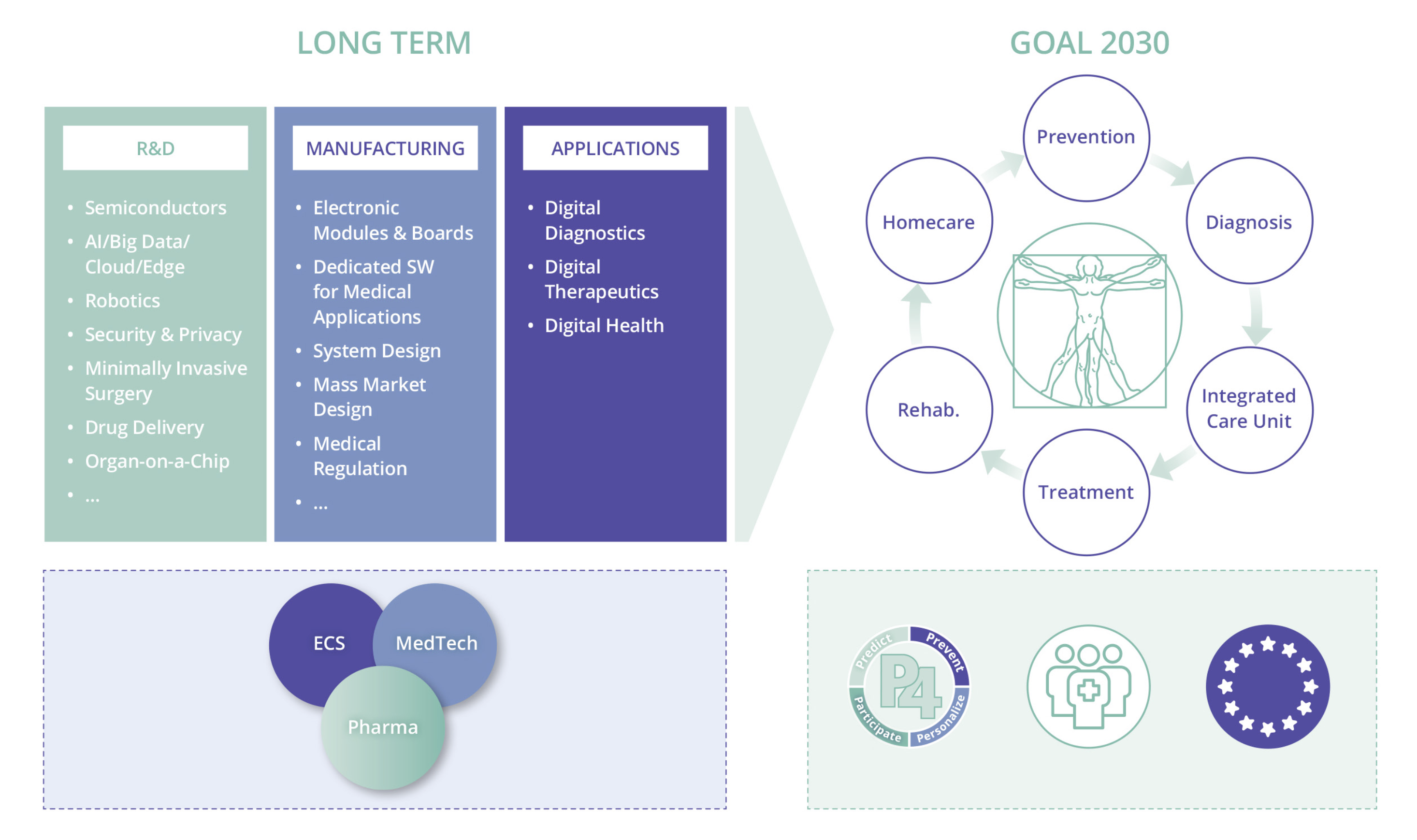
3.4.2.1 Application Trends
Digital health is a relatively new field that is still in its early stages of development.
Wearable devices, remote patient monitoring, prevention, ageing well, P4 medicine are showing great promise for health and wellbeing. The technologies enabled by the chip industry have the potential to significantly disrupt the healthcare industry. They will change the way healthcare is delivered, make healthcare more accessible, and improve patient outcomes.
We already see some effects on projects and proof of concepts, some of them initialised from European public funding. This trend should be amplified for the following reasons.
First, innovation in healthcare is connected to “Industry 4.0”.
“Healthcare 4.0" and "Industry 4.0" are related concepts influenced by different factors specific to their respective industries. Healthcare 4.0 refers to the application of Industry 4.0 technologies specifically within the healthcare sector. The adoption rate of these technologies is slower in health and care due to different regulations, ethical considerations and infrastructure.
“Healthcare 4.0” technologies are fundamentally changing the way healthcare is delivered and received, leading to a more connected, personalised, and data-driven health landscape leading to “integrated care” e.g., the integration of various components of healthcare facilitating coordinated care, multidisciplinary collaboration, personalised medicine...
“Healthcare 4.0” means moving away from centralised clinical models, with a greater focus on patient care outside of hospitals and on behavioural health. “Healthcare 4.0” also has the potential to break down the fragmentation between disciplines. Remote patient monitoring is for instance a cross-cutting application to different pathologies and use cases and opens broader perspectives for manufacturers than the dedicated medical device for a specific pathology. Another correlated and important aspect amplifies this “platform effect”. The boundaries between Pharma, MedTech and Chips companies are blurring due to the digitisation and technologisation of healthcare, e.g., “Healthcare 4.0”. MedTech’s are focusing on the development of advanced wearable devices, implantable sensors, diagnostic tools, and connected healthcare solutions to improve patient monitoring, diagnostics, treatment, and overall healthcare delivery. Pharmaceutical companies are leveraging digital health solutions, such as mobile applications, wearable devices, and remote monitoring systems, to enhance patient engagement, monitor treatment adherence, collect real-time health data, and provide personalised support.
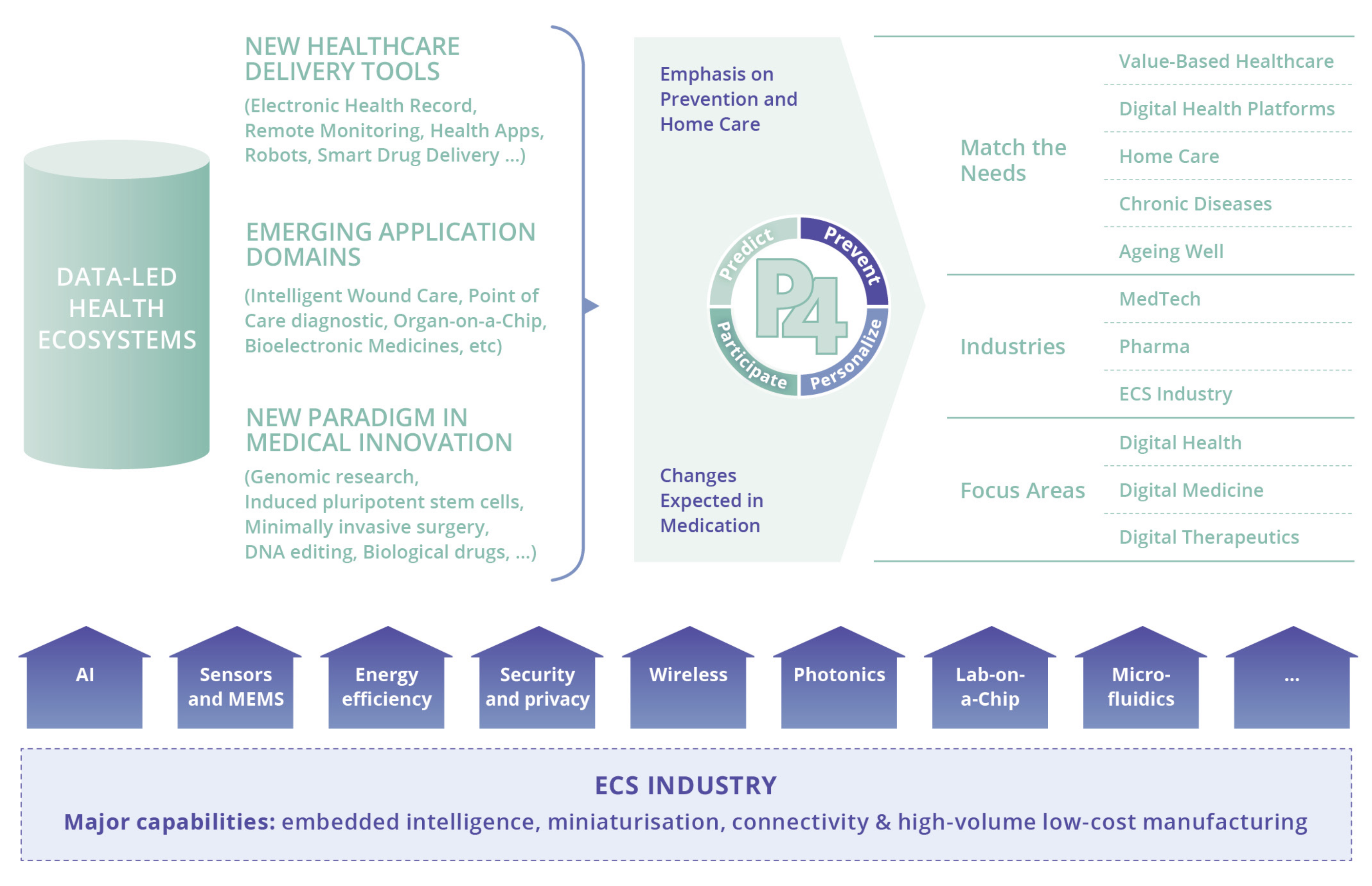
Second, the consumerisation of medical devices leads to an increased demand for and usage of chips. The consumerisation of day-to-day health is tech-driven, with both the involvement of “Big Tech” (Apple Computer, Google, Amazon...) and its most obvious illustration under the form of wearables and consumer apps, is changing the way “Health Consumers” or even “Patients” expect to interact with healthcare.
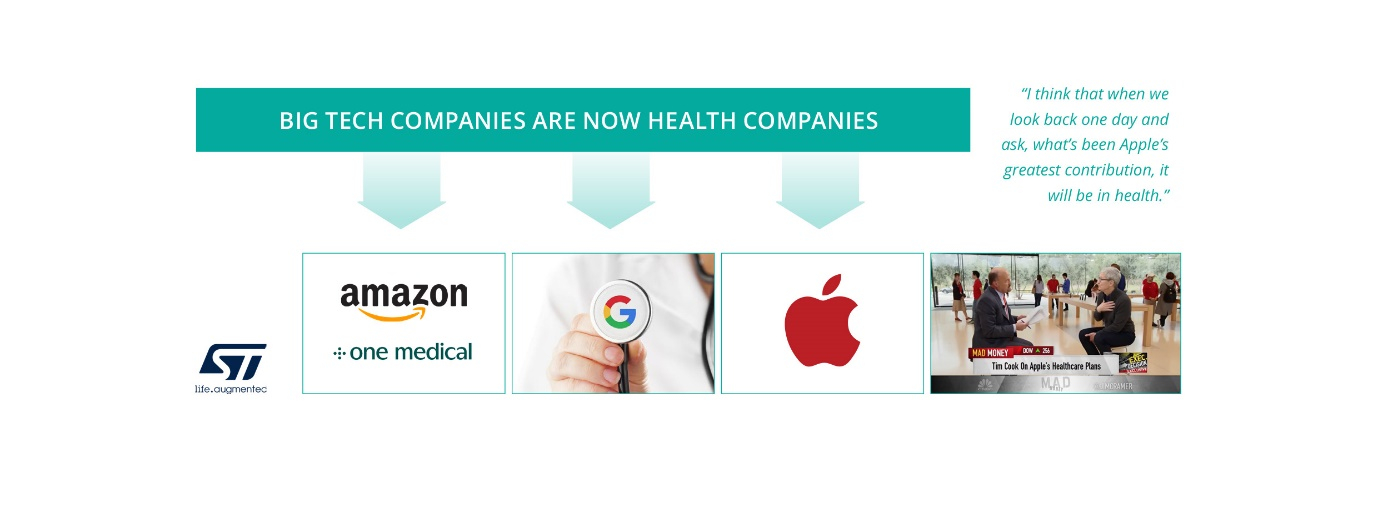
Consumerisation is essential for healthcare systems sustainability, as it emphasises preventive care and early intervention. Consumerisation also encourages innovation and disruptive technologies within healthcare, enabling new care models where patients actively participate in treatment decisions and care plans. Digitisation of healthcare systems is severely lagging the consumer domain and needs reinforcement due to its 'under-the-hood' nature and high complexity and custom requirements, further complicated due to the typically, small product volumes. Consequently, all benefits that we know so well from the consumer domain are missing with many healthcare systems.
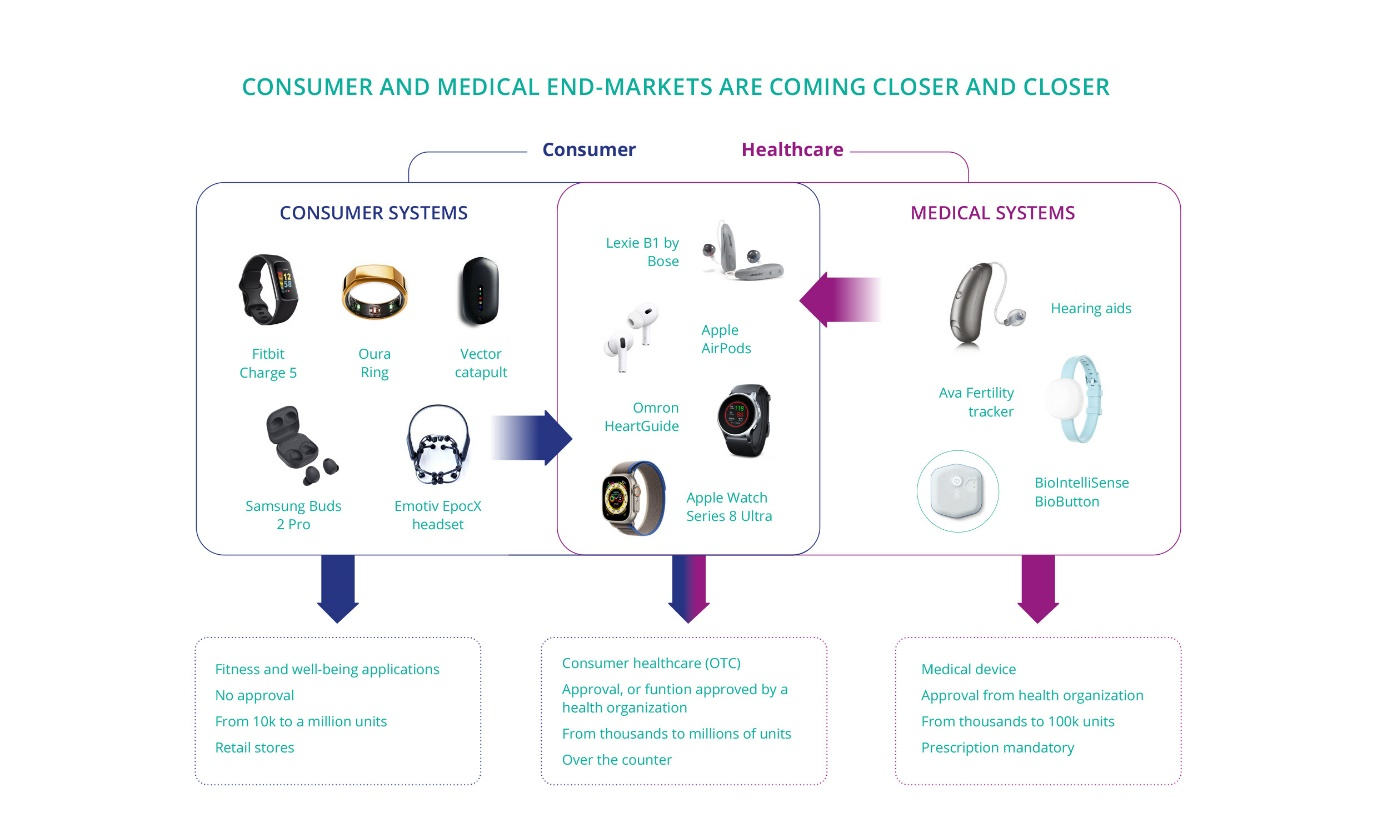
Third, data makes customisation on a large scale possible. Customisation is the driving force behind the trend towards P4 medicine. Mass customisation combines the advantages of mass production (high volume, efficiency, and cost-effectiveness) with the benefits of customisation. Mass customisation in healthcare is closely linked to wearable devices and the Internet of Things (IoT), requires AI and machine learning, allows personalised treatment plans through smart drug delivery systems, and may involve point-of-care diagnostic solutions that enable rapid and personalised testing.
3.4.2.2 Application Trends
Companies in the MedTech and Pharma industries are transitioning from focusing on individual therapies to providing all-inclusive healthcare solutions, develop scalable platforms that can host a range of digital health services utilising smart devices and data analysis to enhance therapy outcomes and align with value-based healthcare principles.
These initiatives will improve the general well-being and quality of life for many people.
Beyond increased accessibility and cost efficiency, incorporating artificial intelligence and advanced data analytics facilitates better collaboration among healthcare professionals to make highly informed decisions, resulting in enhanced patient outcomes. Moreover, the implementation of predictive analytics aids in the timely identification of diseases, promoting early intervention and preventing further complications. Patients have a more interactive and engaged healthcare experience.
Hospitals and clinics can use data analytics to predict patient inflow and manage their resources more efficiently.
Big data analytics can provide valuable insights into disease patterns, treatment outcomes, and patient behaviours, driving research and innovation, and also monitor and respond to global health crises, like pandemics.
A well-known issue in the Digital Health transformation is that regulation must keep pace with innovation. Innovation is potentially limited by a lack of regulations, specifically in innovative areas such as mobile health, digital therapies, and technologies such as big data or AI.
In the European landscape, regulatory hurdles, ecosystem transformation, accurate and redesigned payment schemes are currently addressed to allow the EU to take a strategic advantage in the Healthcare digital transformation.
There are 27 countries in the EU, and 27 health systems.
This fragmentation in the European market for digital health and care exposes innovators to different requirements and processes to achieve market access across the EU. Additionally, patients are limited in using new solutions outside their country of residence.
But there is a political will to develop uses of digital health by moving towards a European single market through a harmonisation of market access. There are currently a bunch of initiatives conducted at European level to enhance the health digital transformation through a European convergence on Digital health and Care.
According to a 2022 “EY” study – “Study on Digital Health implementation in the EU” - almost all countries have established an explicit national Digital Health strategy associated with a roadmap to deploy solutions. The objectives are quite similar:
-
Deployment of core services to the public, either through digital services or by giving access to platforms where health data are stored.
-
Improving patients’ empowerment.
-
The priority service for most European countries is the National Patient Record, which is the core building block and driving force of the digital health strategy.
The creation of a broad “Digital Health Market” beyond the national markets – namely the “Health Digital Single Market” - is a major milestone to overcome the current fragmentation and scale up digital health solutions in Europe. The purpose is to promote and accelerate the adoption of digital health technologies. These technologies are creating a disruption, considering that the life and innovation cycle of digital medical devices are shorter and faster than that of non-digital medical devices. To adjust to this disruption, the usual market access processes for medical devices must be revised. New tools must be implemented to ensure technology acceptance as well as improving health services and research.
-
Data is at the centre of this digital transformation. Currently, the most successful economic business models are almost exclusively data-based platforms and have generated numerous innovations, including in the healthcare sector. These business models promote practices known as “data colonisation”, e.g., promoting the lock-in of valuable data.
Initiatives are conducted in Europe to re-examine the value creation of platform-based business models. The current creation of the European Health Data Space, a unified, interoperable system to share health data illustrates the positive initiatives in the EU healthcare landscape.
-
Reimbursement is a springboard for innovation and must be generalised.
Some countries have now started to establish innovative payment schemes (IPS). They provide a fast-track process for innovative technologies for early market access. It creates a temporary framework that allows innovative technologies to be funded in anticipation of a permanent reimbursement, as it is usually the case with existing market access protocols. Teleconsultation is not part of IPS (this is a digital health service largely reimbursed in the EU).
The 3 main principles of innovation funding are the following:
-
exceptional funding, ahead of coverage by the mainstream health system,
-
limited period,
-
conduct a clinical or medico-economic study.
Acceptance into the innovation funding scheme provides fast-track access to the market. A technology accepted into the innovation funding scheme is usually disseminated across the market via the inclusion of patients in the study, with the assurance that there won’t be any interruption of funding for patients between the clinical study and the request for coverage by the mainstream health system.
According to a survey conducted in 2022 by MTRC across 32 countries, 21 innovative payment schemes were identified in 8 countries (Austria, Belgium, England, France, Germany, Netherlands, Spain, Switzerland). The largest number of schemes were identified in France (n=6), Germany (n=4) and England (n=3).
Beyond these IPS, which are usually covering non-mature solutions, France has introduced common law reimbursement for clinically mature telesurveillance activities, meaning it enables reimbursement by health insurance since July 2023. The scope covers chronic pathologies (heart failure, renal failure, respiratory failure, diabetes, and implantable heart prostheses). France is the first country in Europe to make it happen.
Compared to the Digital Healthcare Act – Digital Health Applications Fast-Track introducing since 2019 apps on prescription in Germany -, the French reimbursement covers the full solution, including wearables or other IoT devices. Another difference lies in the larger coverage regarding the medical device classification, since the reimbursement covers only class I and IIa in Germany, while it is covering all classes in France.
It means overall that progress is made regarding reimbursement and that best practices should be shared all over the EU in a near future.
We summarised the major building blocks of the European digital health market transformation, with some examples of milestones already established or in progress, in the graph below:
-
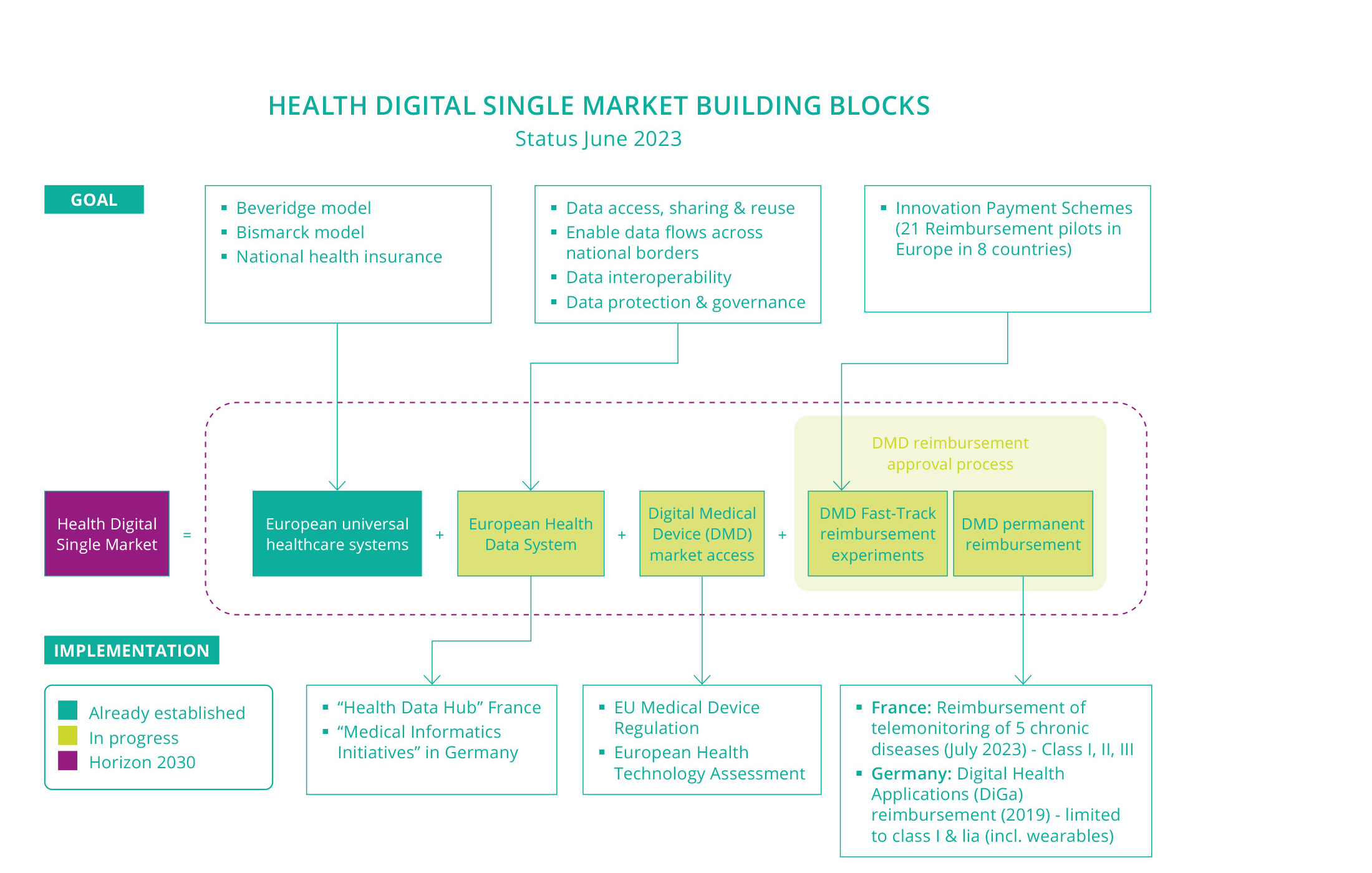
-
European Digital Health use case integration
It is accepted that wearable technology, miniaturised and connected devices embedding intelligence at the edge can serve as valuable tools for prevention, treatment, and management of chronic conditions such as diabetes and heart disease. Their utilisation for prevention and disease management is promised for substantial growth, which explains why the European healthcare system is currently under a major reconfiguration.
What is currently missing, especially from the Chip industry perspective, is scale.
Integrating digital health use cases creates more opportunities for the adoption of connected devices. When digital devices are seamlessly integrated into healthcare workflows and processes, healthcare providers are more likely to incorporate them into their practices. This adoption can drive the volume of connected devices.
Digital health use case integration involves the incorporation of various digital health solutions and applications into healthcare systems to improve patient care, enhance efficiency, and optimise health outcomes.
Understand healthcare customers’ needs requires to collaborate with the hospitals. The hospital is at the centre of the care system. Remote monitoring, home care or ambulatory care are driven by the hospital. Digital health transformation is perceived as a mean to reduce the burden on hospitals and optimise healthcare resource utilisation. This is why it is a great benefit for technology providers to collaborate with entities promoting digital health in the hospital. It is possible to implement frameworks of co-creation, based upon use cases identified by healthcare professionals.
For technology providers, upper in the value chain, an important aspect lies therefore in the end users’ interactions, to understand the use cases where technology can provide an efficient value added, which means (specifically from a technology enabler perspective) avoiding techno-push generating weak return on investments.
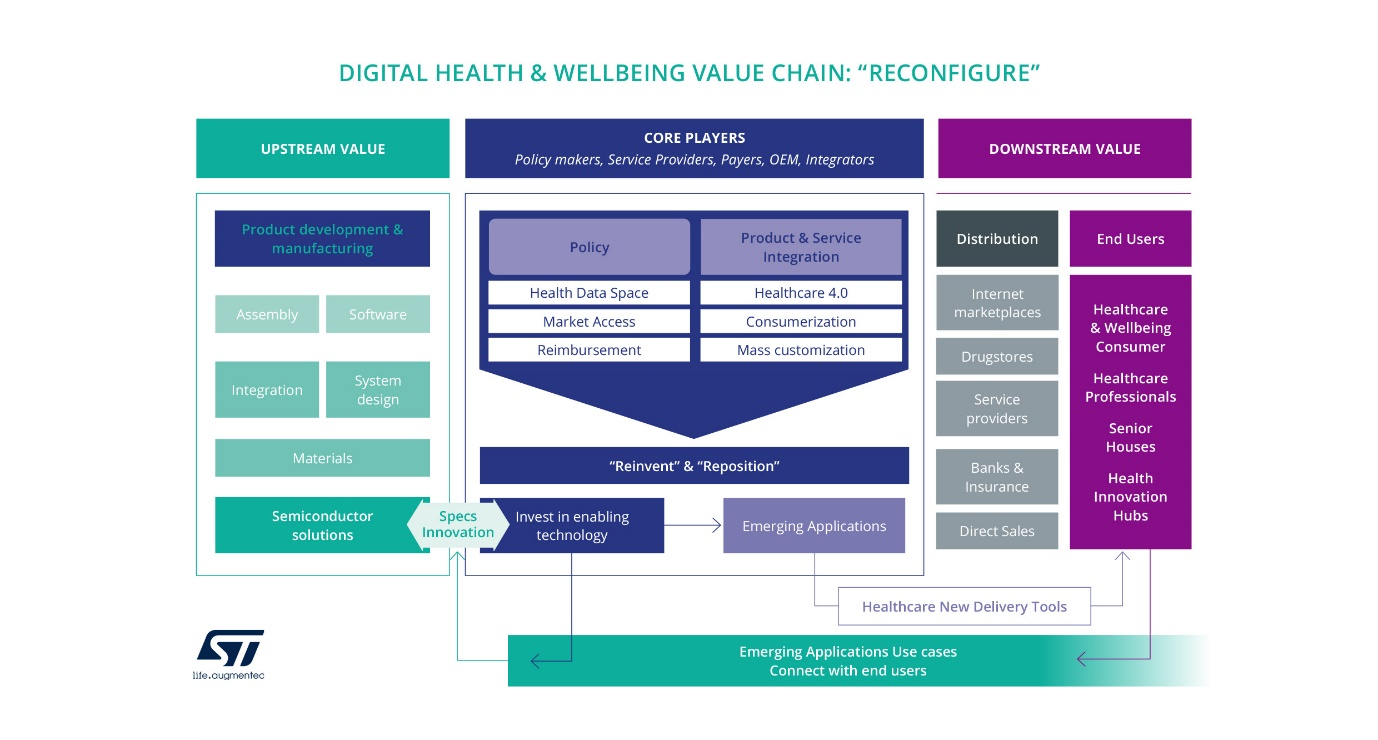
To give EU this strategic advantage, it is essential to converge towards digital health initiatives harmonisation and collaboration among EU countries by fostering the development of common standards, guidelines, technical frameworks, and collaborative projects, allowing the exchange of best practices and knowledge sharing among countries, enabling the implementation of digital health use cases across borders.
As more use cases integrate connected devices, the volume of health data collected increases, leading to a richer dataset for analysis and decision-making.
This shift - leveraging connected medical devices and health data – allowing early detection of health issues, preventive care towards personalised and remote care - is driving the demand that can facilitate the achievement of sustainable healthcare systems across the EU.
Five Major Challenges have been identified for the healthcare and wellbeing domain:
- Major Challenge 1: Enable digital health platforms based upon P4 healthcare.
- Major Challenge 2: Enable the shift to value-based healthcare, enhancing access to 4P’s game-changing technologies.
- Major Challenge 3: Support the development of the home as the central location of the patient, building a more integrated care delivery system.
- Major Challenge 4: Enhance access to personalised and participative treatments for chronic and lifestyle-related diseases.
- Major Challenge 5: Ensure more healthy life years for an ageing population.
3.4.4.1 Major Challenge 1: Enable digital health platforms based upon P4 healthcare
The MedTech industry is in the process of transitioning from an industry primarily producing high-end hospital equipment to one that will increasingly serve point-of-care (PoC) professionals and “health consumers”, thereby moving from a product-based approach to the provision of “integrated services”.
Electronic medical technology, such as the Internet of Medical Things (IoMT), minimally invasive implants, energy-efficient devices, advanced analytics, cognitive computing for advanced clinical decision support, cybersecurity, enhanced network capabilities for continuous data access (to mention only a few of those listed below in the section “Key focus areas”), will support the deployment of P4 healthcare in a data-led environment.
The P4 healthcare vision is therefore not only placing doctors and other health professionals at the centre of the care process, but all those relevant to the health consumer. Even if the healthcare ecosystem is operating in a highly regulated environment, by 2030 we can expect this trend to progressively become the norm. The ECS community should participate in the development of dynamic healthcare systems that learn in real time from every result, achieve a better understanding of treatment response and prognostic heterogeneity, and introduce more refined, patient-tailored approaches to disease detection, prevention, and treatment.
The P4 healthcare vision, enabling for instance early diagnostics based upon merged data and machine-learning techniques through the detection of weak signals, allows preventive treatments that are far less intensive than acute treatments and increase the chances of survival and quality of life. The MedTech industry is not alone on this journey. New pharmaceuticals and treatments will be developed for personalised medicine settings by embedding connected devices and exploiting the potential of the IoT and AI.
This is all creating a new industry, one that revolves around digital health platforms. This platform-based new-market disruption will enable the emergence of specialised platforms, and new players will enter the health domain. This will impact current business models in healthcare, using aggregated data to create value rather than devices – supporting, for instance, proactive services, facilitating outcome evaluation for the treatment of different therapies, and paving the way for outcome-based or pay-per-use reimbursements. This is a potential path to reducing the burden of healthcare expenditures.
The addition of AI capabilities – person-centred AI-based consumer devices/embedded AI-based medical devices and systems – to Smart Things will significantly enhance their functionality and usefulness, especially when the full power of such networked devices is harnessed – a trend that is often called “edge AI”. AI enables much more efficient end-to-end solutions by switching from a centralised to a distributed intelligence system, where some of the analysis carried out in the cloud is moved closer to the sensing and actions.
This distributed approach significantly reduces both the required bandwidth for data transfer and the processing capabilities of cloud servers. It also offers data privacy advantages, as personal source data is pre-analysed and provided to service providers with a higher level of interpretation. It also offers greater reliability and safety.
A high level of digital trust – for privacy and security by design, hardened and embedded AI models – is of course required for executing transactions in healthcare and wellbeing. Securing the IoT ecosystem is a multiple level problem. Privacy should be “by design”. In general, integrating security features into an existing system can become very complex, sometimes impossible, and often increases the cost of the final product significantly. A more efficient approach is to consider those security requirements at the very beginning of a project, and then integrate them in the design and development phase. The ECS industry can assist with end-to-end solutions by providing on-chip security, supplying comprehensive hardware and software services, including authentication, data encryption and access management.
Next-generation connectivity – better performing, more ubiquitous, accessible, secure, and energy-efficient networks – will contribute to unleashing the potential of digital health. One of the main characteristics of future networks will be their increased intelligence to improve the performance of the networks, and offer sophisticated and advanced services to the users, due to edge computing and metadata, for instance.
With the significantly growing number of wearables and other small form-factor, battery-operated devices, very low power consumption is a major technology challenge for product designers. The transition from linear to circular economy will require innovative designs for the lifetime of electronic components and systems, and disruptive changes in ECS supply chains, to reduce the ecological footprint. The ECS industry will contribute to improving energy efficiency – including new, sustainable, and biocompatible energy harvesting – to locally process data and the transmission of pre-processed data as opposed to the transmission of high-volume data (such as imaging data).
As a result of improved integration and analysis of multimodal data, new tools for clinical decision-making and precision medicine will emerge, supporting early diagnostics, personalised medicine and potential curative technologies (e.g. regenerative medicine, immunotherapy for cancer).
3.4.4.2 Major Challenge 2: Enable the shift to value-based healthcare, enhancing access to 4P’s game-changing technologies
A major trend in healthcare is the transformation of large healthcare systems to an optimised hospital workflow: a shift from general hospitals treating any diseases towards integrated practice units that specialise in specified disease types. These units, organised around a medical condition, aim to maximise the patient’s overall outcomes as efficiently as possible, increasingly through remote access, for patients anywhere in the world.
Pay-for-cure rather than pay-for-treatment can be an effective way to increase the efficiency of healthcare by avoiding unnecessary tests, therapies, and prescriptions. Combined with empowered patients, care-givers should be able to make better informed and more effective choices for treatment. To achieve this, outcomes need to cover the full cycle of care for the condition and track the patient’s health status aftercare is completed. This first involves the health status, relying for instance on EHRs supporting precise communication between different care-givers’ PoC diagnostic systems or AI-based clinical decisions. Early diagnosis is key for the successful treatment of both modest and challenging medical conditions.
Health outcomes are also related to the recovery and the sustainability of health. Readmission rates, level of discomfort during care, and return to normal activities should be taken into account for both providers and patients. Humanoid robots applying interpreted human body language and emotion in care delivery, sensors, the deployment of companion devices anticipating and contextualising acute or chronic conditions in EHRs involving health models describing the outcome health values for the patients, both in the short term and long term, will have a direct positive effect on readmission prevention.
To achieve this transformation, a supporting IT platform is necessary. Historically, healthcare IT systems have been siloed by department, location, type of service, and type of data (for instance, diagnostic imaging). An innovative and efficient healthcare information infrastructure – integrating IoT with big data learning for optimising workflow, usage, capabilities and maintenance, and of course digital trust – will aggregate the different areas for efficient value-based healthcare, combining prevention tools, early detection, and treatment. This will enable better measurement and facilitate the design and implementation of new bundle-based reimbursement schemes, reducing costs while improving health outcomes. By 2030, value-based healthcare will enable the adoption of optimisation practices already supported by ECS technologies in the industry.
By 2030, clinical decision-making will be augmented by electronic medical records. Digital centres will enable advanced capabilities for clinical decision-making where AI, real-time data from portable Point-of-Care devices, 3D printing for surgeries, continuous clinical monitoring – including robotics to improve treatments either in the operating room, minimal invasively inside the body, at the general practitioner or at home – will support the integration of specialised care units. A large number of images will be combined with other sensor data and biomedical models to obtain precise, quantified information about the person’s health condition, preventing and providing, for instance, early warnings for (combined) diseases supported by patient health models on complex health conditions. Low–latency, massive image processing is a major information source for AI-based automation, visualisation, and decision support within the whole care cycle. Precise quantified and annotated imaging is needed at many levels: from molecular imaging up to whole body imaging. The development and use of accurate digital twins of the human body will enable clinical trials, individualised computer simulations used in the development or regulatory evaluation of a medicinal product, device, or intervention. While completely simulated clinical trials are not yet feasible, their development is expected to have major benefits over current in vivo clinical trials, which will drive further research on the subject. Moreover, “digital twins” will help in combining all the data on a personal level, and enable personalised clinical decision-making.
Europe is a leading producer of diagnostic imaging equipment. In diagnostic imaging, the ECS industry has begun to place great emphasis on accurate radiation dose monitoring and tracking. Healthcare providers are already applying dose management as part of the quality programme in their radiology departments, and patient-specific computed tomography (CT) imaging and personalisation of scan protocols will be a key aspect of patient-centred care in radiology departments, facilitating the management and control of both image quality and dose with the optimisation of 3D X-ray imaging protocols. In particular, CT and X-ray systems based on the emerging spectral X-ray photon counting technology will enable new ways of imaging in order to reduce dose, improve tissue differentiation, enhance visualisation, or quantify materials.
The enormous capabilities of the ECS industry in miniaturisation, integration, embedded intelligence, communication and sensing will have a major impact on the next generation of smart minimally invasive devices:
-
Highly miniaturised electrical and optical systems realised using advanced cost-effective platform technologies will bring extensive imaging and sensing capabilities to these devices, and enable the second minimally invasive surgery revolution, with smart minimally invasive catheters and laparoscopic instruments for faster and more effective interventions.
-
Sensing and diagnostics solutions need to achieve appropriate sensitivity, specificity, and time-to-result.
-
Reducing waste is possible through sensors made of biological materials, combining a biological component with a physicochemical detector.
-
Fusion of diagnostics and surveillance will help reducing system and operational costs.
-
To realise next-generation smart catheters, a broad spectrum of advanced ECS capabilities will need to be brought together, foremost in dedicated platforms for heterogeneous miniaturisation and integrated photonics. These can be complemented with platforms for embedded ultrasound, low-power edge computing, and AI and digital health platform integration.
-
Optical coherence tomography (OCT) is another example where ECS technologies make a critical impact, in shrinking devices and reducing costs, allowing devices to be used in wider fields beyond ophthalmology.
Finally, it should be noted that the development of the next generation of smart minimally invasive instruments will go hand in hand with the development of new navigation techniques:
-
Breakthrough innovations in photonics are enabling optical shape-sensing techniques that can reconstruct the shape of a catheter over its entire length.
-
MEMS ultrasound technology will enable segmented large-area body conformal ultrasound transducers that are capable of imaging large parts of the body without the need for a trained sonographer, to guide surgeons in a multitude of minimally invasive interventions.
-
Combined with other technologies, such as flexible and conformal electronics, low power edge computing, AI, and data integration into clinical systems, new optical and acoustic- based technologies may eliminate the use of x-rays during both diagnosis and interventions, enabling in-body guidance without radiation.
-
Augmented reality can be used for image-guided minimally invasive therapies providing intuitive visualisation.
As mentioned above, outcomes should cover the full cycle of care for the condition and track the patient’s health status once care has been completed. Biomarkers derived from medical images will inform on disease detection, characterisation, and treatment response. Quantitative imaging biomarkers will have the potential to provide objective decision-support tools in the management pathway of patients. The ECS industry has the potential to improve the understanding of measurement variability, while systems for data acquisition and analysis need to be harmonised before quantitative imaging measurements can be used to drive clinical decisions.
Early diagnosis through PoC diagnostic systems represents a continuously expanding emerging domain based on two simple concepts: perform frequent but accurate medical tests; and perform them closer to the patient’s home. Both approaches lead to improved diagnostic efficiency and a considerable reduction in diagnostic costs:
-
Point-of-care testing (PoCT) methodology encompasses different approaches, from the self-monitoring of glucose or pregnancy, to testing infectious diseases or cardiac problems. However, it should be remembered that disposable PoC devices will need to be environmentally friendly in terms of plastic degradation and the replacement of potentially harmful chemicals.
-
The key enabling components of current PoCT devices must include smart and friendly interfaces, biosensors, controllers, and communication systems, as well as data processing and storage.
-
The emerging lab-on-a-chip (LoC) solutions, embedding multiple sensor platforms, microfluidics, and simple processing/storage elements, are currently the most promising basis for the realisation and development of accurate, versatile, and friendly portable and wearable PoCT devices. Their simplified operation mode eliminates the constraint of molecular biology expertise to perform a real-time reverse transcription polymerase chain reaction (RT-PCR) test, will enable innovative in vitro diagnostic (IVD) platforms, making possible decentralisation from highly specialised clinical laboratories to any hospital lab and near-patient sites, with dedicated sample prep cartridges, a more efficient prevention (referring to the recent Covid-19 pandemic) and prompt personalised diagnosis.
In addition, digital supply chains, automation, robotics, and next-generation interoperability can drive operations management and back-office efficiencies. Using robotics to automate hospital ancillary and back-office services can generate considerable cost and time efficiencies, and improve reliability. Robotic process automation (RPA) and AI can allow care-givers to spend more time providing care. For instance, robots can deliver medications, transport blood samples, collect diagnostic results, and schedule linen and food deliveries – either as a prescheduled task or a real-time request. Robotic processes also can be used for certain hospital revenue cycle and accounting/finance functions, such as scheduling and claims processing.
3.4.4.3 Major Challenge 3: Support the development of the home as the central location of the patient, building a more integrated care delivery system
The trend towards integrated practice units specialising in specific disease types as described in the previous challenge means that certain procedures can move out of the hospital environment and into primary care and home care. Medical equipment that was previously used only in the hospital or clinic is finding its way into the home. For example, tremendous progress has been made since the “consumerisation” of the MEMS in developing compact, accurate, low-cost silicon sensors and actuators. This continuous innovation will support diagnostic and treatment in integrated practice units, while supporting recovery and health sustainability at home. This trend will be supported by the integration of solutions and services for specific disease groups with hospital units to optimise patient-generated health data (PGHD: continuous monitoring, clinical trials at home, etc), enhanced by the integration of heterogeneous devices and systems used at home covering parts of the care cycle (smart body patches, monitoring implants, remote sensing, etc). Solutions are needed that can be integrated into secure health digital platforms, portable end-user devices, remote e-healthcare and AI front-ends.
In addition, the pharmaceutical market is experiencing strong growth in the field of biologics (genomics and proteomics, as well as microarray, cell culture, and monoclonal antibody technologies) that require preparation prior to administration. Smart drug delivery solutions are now based on innovative medical devices for the automated and safe preparation and administration of new fluidic therapies and biologic drugs. These use advanced ultra-low power microcontrollers that control the process reconstitution of the drug based on parameters identified by the practitioner, together with wireless communication modules to transmit data and ensure the patient and treatment are monitored. Smart drug delivery will improve drug adherence as patients will be empowered to administer expensive and complex drugs in their own home.
In this emerging context, care solutions need to be integrated, combining information across all phases of the continuum of care from many sources – preventing, preparing, and providing care based on person-specific characteristics. This will support the development of applicable biomedical models for specific disease groups, for customer groups and for populations, taking heterogeneous data involving history, context, or population information into account.
Supporting prevention, diagnosis and aftercare with sensors and actuators to ensure efficient medical decision, leveraging edge computing and imaging as described in the previous section, will be crucial. The next generation of devices will incorporate increasingly powerful edge computing capabilities. Analysing PGHD from medical devices can be synchronised with a web-based monitoring system. When aggregated, this data can be then sent to the organisation’s health data analytics system to process the results and compare them to previous measurements. If the analysis uncovers negative trends in the patient’s health status, it will automatically notify the care team about possible health risks. The ECS industry can play an important role here in bringing ambulatory monitoring to the next level. The following enabling technology platforms can contribute to this:
-
Low-power technology for sensors, microprocessors, data storage and wireless (microwave, optical, sound) communication modules, etc.
-
Miniaturisation and integration technologies for sensors, microprocessors, data storage, and wireless communication modules, etc.
-
Advanced sensing technologies for multiplex, painless sensing with high sensitivity and reliability.
-
Printed electronics technology for textile integration and the patch-type housing of electronics.
-
Low-power edge AI computing for data analysis and reduction.
-
Data communication technology for interoperability of (wireless) data infrastructure hardware (wearable device connections) and software (data sharing between data warehouses for analysis, and with patient follow-up systems for feedback).
-
Data security technology for interoperability between security hardware and software components (end-to-end information security).
The development of next-generation drug delivery systems will form part of the IoMT – medical devices and applications that link with healthcare systems using wireless connectivity. Smart drug delivery will improve drug adherence so that patients can administer expensive and complex (biological) drugs in their home environment. Enabling platforms are required to facilitate a transition from the legacy mechanical components seen in current autoinjectors and wearable drug delivery pumps, to highly integrated, patch-like microsystems. These include:
-
High-performance sensors and actuators for drug delivery, monitoring and control.
-
On-board microfluidics for in-situ preparation and delivery of formulations.
-
Minimally invasive needles and electrodes for transdermal interfacing, delivery, and diagnostics.
-
New materials, containers and power sources that will meet stringent environmental and clinical waste disposal standards.
-
Body-worn communication technologies for IoMT integration and clinical interfacing.
-
Edge AI for closed-loop control, adherence assessment and clinical trial monitoring.
The development of low-cost, silicon-based MEMS ultrasound transducer technologies is bringing ultrasound diagnostics within the reach of the ECS industry. The ECS industry has the instruments and production technologies to transform these into high-volume consumer products, something no other industry is capable of. Personal ultrasound assisted by AI data acquisition and interpretation will allow early diagnoses in consumer and semi-professional settings, as well as in rural areas. As such, they present a huge opportunity for the ECS industry. It is expected that MEMS ultrasound will enable a completely new industry, with MEMS ultrasound transducers being the enabling platform technology that will drive things on.
Among the emerging applications of advanced MedTech, “smart wound care” – i.e. the merger of highly miniaturised electronic, optical and communications technologies with conventional wound dressing materials – will allow the treatment of chronic wounds of patients in their home without the intervention of daily nursing and/or constant monitoring of the status of the wound. While much progress has been made in wearable technologies over the past decade, new platforms must be developed and integrated to enable the rapid rollout of intelligent wound care. These include:
-
Flexible and low-profile electronics, including circuits, optical components, sensors, and transducers, suitable for embedding within conventional dressings.
-
Advanced manufacturing techniques for reliable integration of microelectronic technologies with foam- and polymer-based dressing materials.
-
Biodegradable materials, substrates, and power sources that will meet stringent environmental and clinical waste disposal standards.
-
Body-worn communications technologies for low-power transmission of wound status.
-
Edge AI to assist the clinical user in data acquisition and data interpretation.
3.4.4.4 Major Challenge 4: Enhance access to personalised and participative treatments for chronic and lifestyle-related diseases
According to the World Health Organisation (WHO) definition, chronic diseases are those of long duration and generally slow progression. Chronic diseases such as heart disease, stroke, cancer, chronic respiratory diseases, and diabetes are by far the leading cause of mortality in Europe, representing 77% of the total disease burden and 86% of all deaths. These diseases are linked by common risk factors, common underlying determinants, and common opportunities for intervention.
One of the crucial means of coping with the prevalence of the chronic diseases is to achieve a more participative and personalised approach, as such diseases require the long-term monitoring of the patient’s state, and therefore need individuals to take greater ownership of their state of health. Most chronic disease patients have special healthcare requirements and must visit their physicians or doctors more often than those with less serious conditions. Technological innovation has already been identified as a great medium to engage chronic patients in the active management of their own condition since digital health offers great convenience to such patients. Access to biomedical, environmental and lifestyle data (through cloud computing, big data and IoT, edge AI, etc) are expected to better target the delivery of healthcare and treatments to individuals, and to tailor each decision and intervention, especially for the treatment of those with multiple chronic diseases.
Patients will be connected seamlessly to their healthcare teams, care-givers and family, as treatment adherence will be more efficient with the innovations mentioned in previous sections. Remote sensing and monitoring offer great promise for the prevention and very early detection of pathological symptoms. Remote sensing and monitoring have the potential to become embedded into everyday life objects, such as furniture and TV sets, while bearing in mind the constraints related to security and privacy. Remote patient monitoring will support clinical decisions with a reduced potential for false alarms, especially for the long-term monitoring and data analysis of patients with chronic diseases.
The ECS industry will need to take the initiative in the development of the next-generation treatment of chronic diseases. The field of remote sensing holds great promise for the lifelong and chronic monitoring of vital signs. The deployment of remote monitoring system relies on sensors integrated into bed or chair. Optical sensing techniques, for instance for remote reflective photoplethysmography, as well as capacitive and radar sensing support this approach. This will be multimodal, with fusing techniques to smart analytics to unify the data into usable information. The strength of remote sensing not only relies on the quality of the acquired signals, but also its potential to reveal slowly changing patterns – possibly symptoms from underlying physiological changes. The analysis of such datasets, currently largely unexplored, will provide new insights into normal versus pathological patterns of change over very long periods of time:
-
Treatment of chronic diseases will be enhanced by an upcoming generation of small and smart implantable neuromodulator devices, which are highly miniaturised, autonomous, and cost-effective. These will be implanted, wirelessly powered by radio frequency (RF), microwave, ultrasound or energy harvesting with minimal side effects on the selected nerve through a simple and minimally invasive procedure to modulate the functions of organs in the treatment of pain management, brain disorders, epilepsy, heart arrhythmia, autoimmune diseases (immunomodulation), etc.
-
Organ-on-a-chip (OOC) platforms, which lie at the junction of biology and microfabrication and biology for personalised and safer medicines, are another treatment approach, addressing, for instance, pathologies currently without effective treatment (rare diseases). Often rare diseases are chronic and life-threatening, and they affect approximately 30 million people across Europe. In an OOC, the smallest functional unit of an organ is replicated. The essential capabilities underlying the OOC field are primarily embedded microfluidics and the processing of polymers in a microfabrication environment. Smart sensors can be used as readout devices, while edge AI will be essential in data interpretation and reduction.
For chronic diseases diagnoses, LoC-based technologies – relying on miniaturisation – show promise for improving test speed, throughput, and cost-efficiency for some prominent chronic diseases: chronic respiratory diseases, diabetes, chronic kidney diseases, etc.
3.4.4.5 Major Challenge 5: Ensure more healthy life years for an ageing population
In the last two decades, effort has been made to enhance two important and specific objectives of smart living environment for ageing well:
-
Avoid or postpone hospitalisation by optimising patient follow-up at home.
-
Enable a better and faster return to their homes when hospitalisation does occur.
The following list includes some typical examples of assistance capabilities related to major chronic diseases covering the first main objective (optimisation of patient follow-up at home):
-
Vital signs checker: blood pressure meter, oximeter, thermometer, weight scale.
-
Hospital's software interface, the patient’s file, the patient's risk alarm centre with automatic call to healthcare practitioners.
-
Video communication support (between the patient and their nurse, doctor, and family), and interactive modules for the patient (administrative, activities, menus, medical bot chat, etc.).
-
Authentication and geolocation of patients, with patient or patient’s family consent.
-
Teleconsultation for nights and weekends at the foot of the bed of patient’s hospital or retirement home.
The second objective – smooth home return – relates to suitable technical assistance in addition to human assistance:
-
Enhance the patient's quality of life and autonomy.
-
Improve the patient's safety and follow-up in their room through a reinforced work organisation.
-
Allow monitoring of the patient’s progress to motivate them during their rehabilitation period.
-
Minimally invasive therapies allowing for shorter hospital stays and improved patient wellbeing.
-
First-time-right precision diagnoses to prevent hospital readmissions.
-
Prepare for the return home: patient support in appropriating technical aids by integrating these solutions into rehabilitation.
Efforts are being made to enhance medical and social care services through different kinds of robots. The purpose here is to provide advanced assisted living services via a general purpose robot as an autonomous interaction device that can access all available knowledge and cooperate with digital appliances in the home. In this sense, autonomous mobile robots offer several advantages compared to the current (stationary) Ambient Assisted Living (AAL) solutions. Due to sensor-augmented user interfaces, human computer interaction is becoming increasingly natural. As a consequence, robots will come to represent a familiar metaphor for most people.
Neurorehabilitation is sometimes required after hospitalisation and is generally a very complex and challenging undertaking resulting in both “successes” and “failures” (setbacks). Neurological patients typically report having “good days and bad days”, which affect performance, motivation and stamina, and where cognitive stimulation (AI-based speech producing programs, social robots, etc), for example, has the potential to improve the efficiency of neurorehabilitation and relieve some of the pressure on health systems. Robotics is well suited for precise, repetitive labour, and its application in neurorehabilitation has been very successful. This is one of the main reasons why the rehabilitation robotics market has tripled over the last five years and, today, rehabilitation robotics is one of the fastest growing segments of the robotics industry. This industry is dominated by European companies that can deliver highly innovative solutions with a strong scientific basis and exceptional manufacturing quality. Based on market size and need, it is projected that the compound annual growth rate (CAGR) for rehabilitation robotics will soon reach between 20% and 50%.
The ECS industry can significantly upscale the “ageing well” area, as it is enabled by most of the focus topics developed in the previous sections. The industry is playing an important role in bringing ambulatory monitoring to the next level. Important aspects here are reducing costs, improving user friendliness (e.g. easy to wear/use devices, interoperable gateways, reduction of patient follow-up systems) and data security.
The enabling technology platforms detailed below are expected to significantly contribute to this prevalence of the ECS industry in ageing well, taking into account that ageing well is very much related to “ageing in place”:
-
Low-power technology for sensors, microprocessors, data storage and wireless communication modules, etc.
-
Miniaturisation technology for sensors, microprocessors, data storage and wireless communication modules, etc.
-
Printed electronics technology for textile integration and patch-type housing of electronics.
-
Low-power edge AI computing for data analysis and data reduction.
-
Data communication technology for interoperability of (wireless) data infrastructure hardware (wearable device connections) and software (data sharing between data warehouses for analysis and with patient follow-up systems for feedback).
-
Data security technology for interoperability between security hardware and software components.
-
Robotics systems enabling patients to overcome loneliness or mental healthcare issues.
Interoperability is surely the main challenge faced by the ECS industry in achieving full impact due to the vast heterogeneity of IoT systems and elements at all levels. Interoperability and standardisation need to be elaborated in relation to data and aggregated information. Thus, it is not enough to be able to receive a message, i.e. to understand the syntax of the message, but it is also necessary to understand the semantics. This requirement implies the development of a data model that maps semantic content from the data received from devices into an information system that is usually utilised for collecting and evaluating data from monitored persons. It must be based on several relatively simple principles: creation of formats and protocols for exchange of data records between healthcare information systems; format standardisation and connected interface unification; improvement of communication efficiency; a guide for dialogue between involved parties at interface specification; minimisation of different interfaces; and minimisation of expenses for interface implementation.
The following table illustrates the roadmaps for Health and Wellbeing.
MAJOR CHALLENGE | TOPIC | SHORT TERM (2024–2028) | MEDIUM TERM (2029–2033) | LONG TERM (2034 and beyond) |
---|---|---|---|---|
Major Challenge 1: Enable digital health platforms based upon P4 healthcare |
Topic 1: establish Europe as a global leader in personalised medicine deployment |
|
|
|
Major Challenge 2: lead the healthcare system paradigm shift from treatment to health promotion and prevention |
Topic 2: enable the shift to value-based healthcare |
|
|
|
Major Challenge 3: home becomes the central location of the “healthcare consumer” |
Topic 3: build an integrated care delivery system |
|
|
|
Major Challenge 4: ECS industry supports EU strategy to tackle chronic diseases |
Topic 4: enhance access to personalised and participative treatments for chronic and lifestyle-related diseases |
|
|
|
Major Challenge 5: ECS industry fosters innovation and digital transformation in active and healthy ageing |
Topic 5: ensure more healthy life years for an ageing population |
|
|
|
Close collaboration will be useful in all application areas – for example, Energy, Mobility, Digital Industry, Agrifood and Natural Resources, and Digital Society – based on cross-sectional technologies such as Edge Computing and Embedded Artificial Intelligence, Connectivity and, of course, Quality, Reliability, Safety and Cybersecurity.
More specifically:
-
Related to digital industry, “bio-production”, which has the objective of developing an innovative field to produce the biologic products of the future through the implementation of disruptive technologies, should be an important topic to address in future years.
-
The relationship between food systems and health is obvious and well-identified, especially in preventive health. This is an aspect that needs to be followed to reinforce health prevention in the long term.
-
In terms of energy and connectivity, it is important to consider the impact of innovative wearables and implantables, sensors and actuators in general, as they represent a crucial sector with a direct impact on the further development of digital health.
-
Embedded systems are an essential enabler of healthcare digital transformation. Medical systems have special requirements regarding hardware quality and reliability, dependability in connected software and human/ systems interaction. Also, privacy and cybersecurity are required to support the expansion of digital health. Related challenges are defined in the transversal Chapter Quality, Reliability, Safety and Cybersecurity.