2 CROSS-SECTIONAL TECHNOLOGIES
2.2
Connectivity
Connectivity and interoperability technologies in ECS enabling business and social benefits are tied to layers 1, 5, and 6 of the OSI model. The focus on these layers is motivated by the Major Challenges that characterize them (see Figure 2.2.1).
The scope covers the following types of physical layer connectivity.
-
Cellular:
-
Beyond 5G
-
6G
-
Direct to cell (Supplemental Coverage from Space scenario)
-
-
Satellite communication
-
Low Earth Orbit and geosynchronous equatorial orbits
-
Non Terestrial Network
-
-
Low power wide area:
-
Cellular: narrow band IoT, LTE, 6G, etc.
-
Non-cellular: SigFox, LoRa, M-Bus, etc.
-
-
Low power short range:
-
Wireless: existing (Bluetooth, WiFi, etc.) or innovative technologies (mmW, etc.).
-
Wired: covering both high-speed optical glass and plastic fibers, mmW plastic fibers, and copper interconnect (USB, DOCIS, PCIe, UCIe, AIB, etc.).
-
-
High speed:
-
Wireless: point to point mmW .
-
Wired: high-speed optical (800 Gb+, etc.) and copper interconnect (Ethernet, etc.).
-
The main challenge will be to ensure European leadership in terms of connectivity technologies (for example, standards) as well as associated software and hardware technologies supporting the development of connectivity solutions (e.g. chipset, module, protocols, etc.).
The scope addressed in this context is the interoperability from application to application relying on technologies at OSI layers 5 and 6. This interoperability covers the following underlying aspects:
-
Protocols at all technology levels: Internet, operational and legacy.
-
Security: such as protocol security, payload encryption, authentication, authorization, certificates, tokens and key distribution.
-
Data semantics: supporting application to application understanding of transferred data/information.
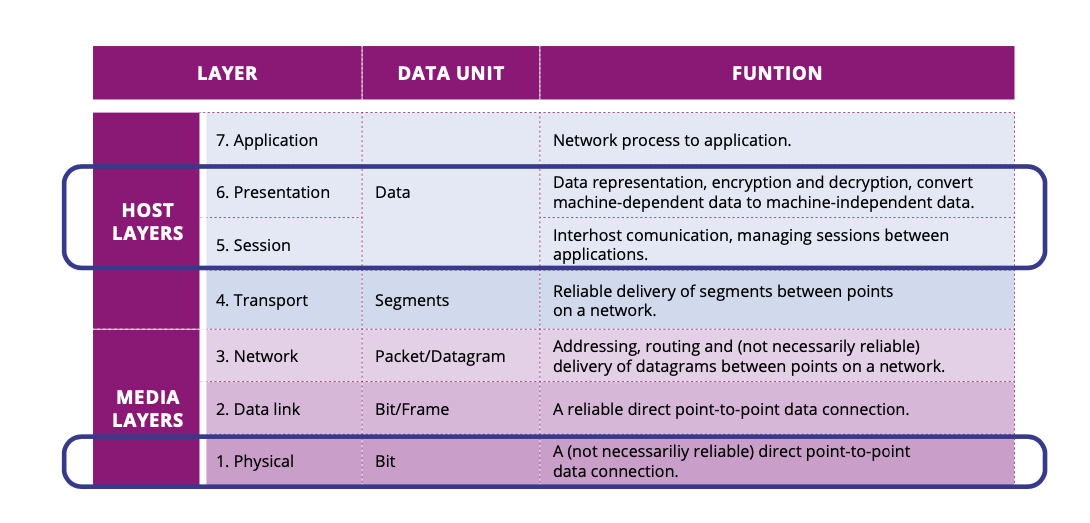
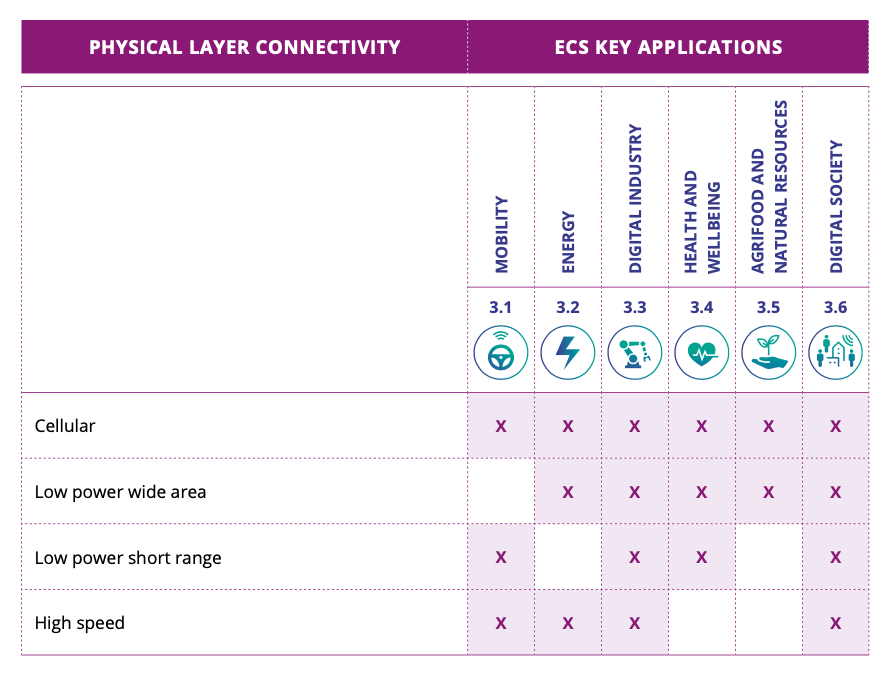
Improvements in connectivity technology will have an impact on all ECS application areas. For health and well-being, connectivity interoperability issues are addressed by enabling faster translation of ideas into economically viable solutions, which can be further scaled up in daily health practice. Examples of health and well-being application breakthroughs supported here are:
-
A shift in focus from acute, hospital-based care to early prevention.
-
Strengthening where and how healthcare is delivered, supporting home-based care.
-
Stronger participation of citizens in their own care processes, enhancing patient engagement.
-
Supporting the clinical workforce and healthcare consumers to embrace technology-enabled care.
-
Data communication technology for interoperability of wireless data infrastructure.
Heterogeneous and chiplet integration connectivity enabling European research, prototyping and large scale production addressing market needs in key application domains like e.g. automotive (SDV/Risc-V), HPC, industrial.
Improved, secure and interoperable connectivity will further support healthcare and well-being application breakthroughs regarding, for example:
-
Healthcare deployment, enabling digital health platforms.
-
Healthcare system paradigm transition from treatment to health prevention, enabling the shift to value-based healthcare.
-
Building a more integrated care delivery system, supporting the development of the home as the central location for the patient.
-
Enhancing access to personalized and participative treatments for chronic and lifestyle- related diseases.
-
Enabling more healthy life years for an ageing population.
In the mobility application area, the provision of improved, robust, secure and interoperable connectivity will support breakthroughs regarding:
-
Achieving the Green Deal for mobility, with the 2Zero goals of –37.5% CO2 by 2030.
-
Increasing road safety through the CCAM program.
-
Strengthening the competitiveness of the European industrial mobility digitization value chain.
In the energy application domain, the provision of improved, robust, secure and interoperable connectivity will support breakthroughs regarding:
-
Significant reduction of connectivity energy demand.
-
Enabling necessary connectivity to the integration of the future heterogeneous energy grid landscape.
-
“Plug and play integration” of ECS into self-organized grids and multi-modal systems.
-
Solving safety and security issues of self-organized grids and multi-modal systems.
In the industry application domain, the provision of improved, robust, secure and interoperable connectivity will support closing gaps such as:
-
Preparing for the 5G era in communications technology, especially its manufacturing and engineering dimension.
-
Long-range communication technologies, optimized for machine-to-machine (M2M) communication, a large number of devices and low bit rates, are key elements in smart farming.
-
Solving IoT cybersecurity and safety problems, attestation, security-by-design, as only safe, secure and trusted platforms will survive in the industry.
-
Interoperability-by-design at the component, semantic and application levels.
-
IoT configuration and orchestration management allowing for the (semi)autonomous deployment and operation of large numbers of devices.
In the digital society application domain, the provision of improved, robust, secure and interoperable connectivity will support the overall strategy regarding:
-
Enabling workforce efficiency regardless of location.
-
Stimulating social resilience in the various member states, providing citizens with a better work/life balance and giving them freedom to also have leisure time at different locations.
-
Ubiquitous connectivity, giving people a broader employability and better protection against social or economic exclusion.
-
Enabling European governments, companies and citizens to closer cooperation, and to develop reliable societal emergency infrastructures.
In the agrifood application domain, the provision of improved, robust, secure and interoperable connectivity will support innovations addressing the EU Green Deal regarding:
-
Reducing the environmental impact related to transport, storage, packaging and food waste.
-
Reducing water pollution and greenhouse gas emission, including methane and nitrous oxide.
-
Reducing the European cumulated carbon and cropland footprint by 20% over the next 20 years, while improving climatic resilience of European agriculture and stopping biodiversity erosion.
Five Major Challenges have been identified in the connectivity domain:
-
Major Challenge 1: strengthening the EU connectivity technology portfolio to maintain leadership, secure sovereignty and offer an independent supply chain.
-
Major Challenge 2: investigate innovative connectivity technology (new spectrum or medium) and new approaches to improving existing connectivity technology to maintain the EU’s long-term leadership.
-
Major Challenge 3: autonomous interoperability translation for communication protocol, data encoding, compression, security and information semantics.
-
Major Challenge 4: architectures and reference implementations of interoperable, secure, scalable, smart and evolvable IoT and SoS connectivity from edge to cloud
-
Major Challenge 5: network virtualization enabling run-time and evolvable integration, deployment and management of edge to cloud network architectures.
2.2.3.1 Major Challenge 1: Strengthening the EU connectivity technology portfolio to maintain leadership, secure sovereignty and offer an independent supply chain
Today’s connectivity solutions require an incredibly complex electronic system comprising various functions integrated into a wide range of technologies.
Note that advanced digital functions such as the application processor and the baseband modem are mastered by a limited number of US and Asian players (Mediatek, Qualcomm and Samsung), and achieved in advanced complementary metal–oxide–semiconductor (CMOS) technology available at only two Asian businesses (Taiwan Semiconductor Manufacturing Company, TSMC, and Samsung). On this last point, it is worth noting that through the America CHIPS Act, Intel, SAMSUNG and TSMC will build new advanced logic (3 nm and below) 300 mm Fabs in Arizona to strengthen US sovereignty and supply chain.
The European Chips Act supports ST and GF new 300 mm facility in France build to allow for 18 nm and beyond FDSOI technology manufacturing (while <10 nm R&D activities are pursued both at CEA Leti and IMEC), all this in order to limit the reliance on Asian foundries’ manufacturing capabilities. Consequently for the foreseeable future, Europe most advanced semiconductor manufacturing capability will be limited to 18/16 nm CMOS node which a consequence of the products currently designed by European semiconductor players and required by associated key verticals (automotive, industrial, ...). This point is also illustrated by the decision of TSMC to start building during summer 2024 a new 16/12 nm 300 mm Fab in Dresden (11B $ investment) through the creation of a joint venture working with Infineon, NXP, and Bosch (each holds a 10% stake)
In fact, European players (Infineon, NXP, ST, etc.) are strong on the analogue and RF front end module markets, mainly due to the availability of differentiated technologies developed and manufactured in Europe. Differentiated technologies are a key strength of the European ECS industry, especially when considering the connectivity market. Consequently, to maintain Europe’s leadership and competitiveness it is vital to ensure that European differentiated semiconductor technologies remain as advanced as possible. This is key to ensure that Europe secures the market share in the connectivity market, and also strengthens its technology leadership by playing a major role in the development and standardization of future connectivity technologies. This point is crucial to secure Europe’s sovereignty on the connectivity topic.
Moreover, over the last year the rising economic tension between the US and China has underlined the value of Europe's ECS supply chain. Once again, this is especially true for differentiated technologies. For example, advanced BiCMOS technologies are currently mastered by a limited number of US (GlobalFoundries and TowerJazz) and European (Infineon, ST and NXP) players. With Chinese companies being forced to move away from US providers, this creates a significant opportunity for Europe as the only viable alternative. Consequently, strengthening Europe’s connectivity technology portfolio and associated manufacturing capacity to offer an independent and reliable supply chain is now a key challenge for all European ECS actors.
In addition to being able to provide the differentiated semiconductor technologies supporting the development of innovative connectivity solutions, it is important to note that some European players are proposing connectivity chipset solutions (for example, Sequans Communications and Nordic on the narrowband IoT topic) or full connectivity solutions. Supporting the growth of these existing actors and helping emerging industry leaders is also a key challenge for Europe to capture a bigger proportion of the value chain, as well as to ensure its sovereignty on the connectivity topic in the long run.
To address identified connectivity technology challenges, we propose the vision described below, which can be summarized by the following three key points (with associated expected outcomes).
Strengthening Europe’s differentiated technologies portfolio
As discussed above, Europe’s differentiated semiconductor technologies are key assets that should be both preserved and improved upon to secure European leadership in connectivity. Consequently, dedicated research should be encouraged, such as the technologies below (which are also promoted in the Chapter 1.1 on Process Technology, Equipment, Materials and Manufacturing):
-
Advanced BiCMOS: targeting RF and sub-THz (i.e., 100-300GHz) and THz front-end modules.
-
RF SOI: targeting <7 GHz and mmW front-end modules.
-
GaN and Gan on Si: targeting the high-power infrastructure and high efficiency/wide bandwidth 6G handset markets (5.925 GHz – 7.125 GHz and 10 GHz – 13.25 GHz bands).
-
FD SOI: targeting power-efficient connectivity solutions (for example NB IOT and NTN).
-
GaAs: targeting mmW space and defense applications ( W & K bands).
-
InP & InP on Si: targeting high-speed optical link (>800 Gb/s), mmW applications (6G sub-THz communication >100 GHz) and ultra-low noise Front End Module in K band.
-
Silicon Photonics: targeting next generation silicon photonics technology (SiN waveguide, thin film LNO, ...) to address both pluggable optics for data centre and AI driven co-package optics solution
-
RF filters: supporting the development on innovative European based technology (for example POI based TF SAW technologies).
-
Advanced packaging: enabling prototyping and medium volume production capability in Europe to enable heterogeneous integration of differentiated technology manufacture in Europe to move higher in the value chain and capture more value.
The main challenge will be in to improve achievable performances. To illustrate this, we have extracted the medium-term (2025) and long-term (2030) solid state technology roadmap proposed by H2020 CSA project NEREID to serve as a connectivity roadmap (see Figure 2.2.3). We can see that whatever the type of application (device-to-device, D2D, indoor, outdoor), the requirements in analogue RF will mainly consist of achieving Fmax and FT ~500 GHz in 2025 and 1 THz in 2030, while NFmin will be well below 1 dB in the medium term, to reach 0.5dB in the long term. The only parameter that differentiates the types of applications is the output power, which outdoors should reach between 36 and 40 dBm per PA by the end of the decade. The biggest challenge for silicon or hybrid-on-silicon substrate technologies is expected to be the frequency challenge. Technologies such as GaN/Si and RF SOI will deliver power but for applications operating at less than 100 GHz.
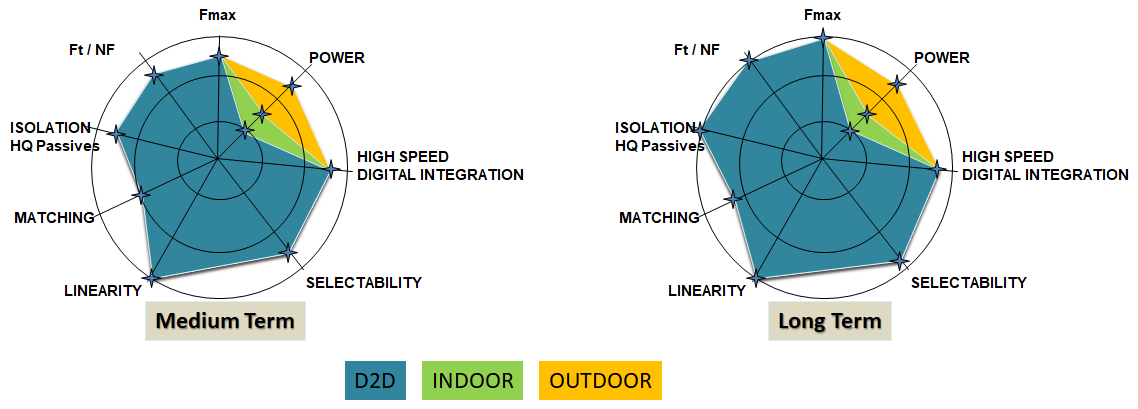
Note that the vision presented in Figure 2.2.3 also applies to packaging and printed circuit board (PCB) technologies. It is also worth noting that while Europe is playing a key role in innovative differentiated semiconductor technologies, there is very little R&D activity or few players in Europe on the packaging and PCB side. This point is clearly a weakness that should be addressed to strengthen Europe’s connectivity technology portfolio.
Securing Europe’s differentiated hardware technology manufacturing
Beyond the development and enablement in Europe of innovative semiconductor technologies targeting the connectivity market, it will be key to safeguard and promote European manufacturing capability to both secure Europe economical interest (in terms of market share) and also address the sovereignty topic (since trade war issues can jeopardize the viability of Europe’s industrial actors). To do so, in coordination with chapter 1.1 on Process Technology, Equipment, Materials and Manufacturing, the following topics should be supported:
-
The enablement of pilot lines: the objective here is to support the deployment of additional manufacturing capabilities for technology already available in Europe (supporting the transition to 300 mm Fab), or to address new technologies (such as packaging or advanced PCB) to increase the technology portfolio available in Europe.
-
The rise of new semiconductor equipment champions: to secure manufacturing capabilities in the long term, it will also be necessary to ensure that the required equipment is provided by European players. This is crucial to prevent any vulnerability in the European supply chain to possible international political or economic issues.
-
Nurture a pan-European design ecosystem to tackle the new challenges on transistors in more than Moore, circuits architectures with increased security and trustworthiness and new AI/ML chips raised by the digitization era.
Strengthening Europe’s connectivity technology portfolio (hardware, internet protocols and software)
Leveraging previously discussed differentiated semiconductor technology portfolio, innovative connectivity solutions (hardware, internet protocol software) should be encouraged to enable Europe to take full advantage of its technology and manufacturing assets, and to capture market share at the component level. This action is crucial to secure Europe's position beyond 5G and 6G standardization and preliminary deployment activities. It also enables the development and manufacturing in Europe of highly integrated connectivity module/systems.
Since most of the value of a complex connectivity system will be captured at the module level, it is highly desirable to enable European players to rise in the value chain (in coordination with the chapter Components, Modules and Systems Integration).
In targeting systems and applications, it is important to consider the interconnection between subsystems, and focus should be on individual component technology development according to needs identified at the system or application level. To support this system vision, the promotion of innovative technology enabling heterogeneous and chiplet integration is key.
Heterogeneous and chiplet integration refers to the integration of separately manufactured components into a higher- level assembly that cumulatively provides enhanced functionality and improved operating characteristics. In this definition, components should be taken to mean any unit – whether individual die, device, component, and assembly or subsystem – that is integrated into a single system. The operating characteristics should also be taken in their broadest meaning, including characteristics such as system-level cost of ownership.
This is especially true for the hardware side in the context of the end of Moore’s law. It is the interconnection of the transistors and other components in the integrated circuit (IC), package or PCB and at the system and global network level where future limitations in terms of performance, power, latency and cost reside. Overcoming these limitations will require the heterogeneous integration of different materials (silicon, III-V, SiC, etc.), devices (logic, memory, sensors, RF, analogue, etc) and technologies (electronics, photonics, MEMS and sensors).
To support the vision presented in the previous paragraph, we propose to focus effort on the following key focus areas:
-
Materials (GaN, InP, etc.) and large diameter wafers technology (POI, InP on Si, GaN on Si, InP etc.) supporting the development of innovative connectivity technology solution.
-
Differentiated semiconductor technology development targeting connectivity application.
-
Packaging and PCB technology targeting connectivity application.
-
Pilot line enablement to support the strengthening of European manufacturing capability.
-
Connectivity solution engineering through virtualization of the different connectivity layers.
-
Connectivity for high speed low energy Heterogeneous and chiplet integration
-
Ultra‐low power transceivers with low eco‐footprint.
-
Power efficient and cost efficient transceivers including Data conversion (ADC-DAC), up & down frequency conversion (LO & mixers), and RF emission and Reception (PA-LNA).
-
Advanced System on Chip design for CMOS and new technologies like e.g. GaN, InP, InP on Si, GaN on Si.
-
Innovative EDA solutions leveraging AI capability.
-
The enablement of a EU fabless ecosystem which could leverage advanced semiconductor manufacturing in Europe and enable to strengthen EU position in the value chain.
2.2.5.2 Major Challenge 2: Investigate innovative wireless connectivity technology (new spectrum or medium) and new approaches to improving existing connectivity technology to maintain the EU’s long-term leadership
Targeting connectivity solutions beyond 5G, R&D activity is today mainly focused on the three key challenges listed below.
Evaluating the advantage to use new spectrum (especially 6 GHz – 15 GHz band and mmW frequencies >100 GHz)
With the ongoing deployment of 5G in the <6 GHz, the current R&D focus is now focused on the spectrum considered for 6G development targeting the 2030 time horizon. Three main frequency bands are today discussed in the telecommunication industry (following the recent decision of WRC23 in December 2023):
Low band (6.425 GHz – 7.125 GHz):
One of the significant outcomes of WRC-23 was the allocation of the 6.425 GHz – 7.125 GHz band as a licensed one dedicated to 6G while the lower 6 GHz spectrum (5.925 GHz – 6.425 GHz) will remain available for unlicensed usage (namely Wi-Fi). WRC-23 thus gave a balanced decision which supported both services. We can note that while this new spectrum will be the main 6G one identified by most region to start deployment in the 2030 time frame, the USA has adopted a complete opposite position. While China is considering to even allocate the lower 6 GHz spectrum for IMT services, the US did the opposite and the FCC allocated the full 5.925 GHz – 7.125 GHz band for Wi-Fi (raising the question of which low frequency spectrum may be used to deploy 6G in the USA). Development of wireless system in the 6.425 GHz – 7.125 GHz will likely be incremental to previous one <6 GHz, however we can mention that PA efficiency (GaN opportunity on the handset market?) and filtering technology (due to coexistence issues with Wi-Fi) seem to be the main challenges today.
Mid band (7.125 GHz – 8.4 GHz):
WRC-23 also set the agenda for the next World Radiocommunication Conference in 2027 (WRC-27) with a clear roadmap for future IMT spectrum allocations. One of the key identified new spectrum to be evaluate is the 7.125 GHz - 8.4 GHz one since it seems to be the most likely solution to add new spectrum for 6G deployment in the US (a federal spectrum study working group started in march 2024 with the goal of releasing a final report on potential commercial access to the band by October 2026).
Since it may prove difficult to clear this entire spectrum from existing usage, we can note that the 7.75 GHz – 8.4 GHz band is attracting a lot of attention since such scenario poses less technical difficulty and has the advantage to be considered by other region than the USA.
From hardware technology point of view, working over 8 GHz will bring several challenges. From PA side, we can wonder if GaAs HBT technology will remain relevant. From filter side, It is not clear if existing filter technologies will provide acceptable performances. More generally, it creates an opportunity for SiGe technology (and consequently for Europe) since this technology is today widely used in the X and Ku bands for satellite communication.
NTN in Ku & Ka bands:
In addition to new spectrum requirements previously mentioned, 6G envision to seamlessly integrate existing satellite communication in cellular connectivity networks. This trend can be seen under the topic Non Terrestrial Networks currently discussed inside 3GPP.
Targeted frequency spectrum is not new (traditional Ku and Ka bands). The main objective is here to leverage under deployment Low Earth Orbit satellite constellation to complement the cellular network coverage while offering performances (latency & data rate) in line with real-time application.
Two main challenges will have to be addressed: seamless integration and handover of satellite connectivity along with cellular one (network type should be transparent for the user) and the development of cost-effective chipset solutions to enable user terminals in line with mass market constraints (which creates key opportunities for Si-based technology such as SiGe BiCMOS).
We can note that on this NTN topic, Supplemental Connectivity from Space is a new trend to be kept in mind. The idea consists in reusing frequency bands used by cellular operators on the ground to complement their existing cellular network with a LEO satellite connectivity. The main ongoing development concerns what SpaceX and T-Mobile are doing in the US. The advantage is here to leverage an installed base of user’s equipment (4G smartphones) since the only new hardware is from the infrastructure side (the LEO satellite constellation). This development is today limited to what regulator are authorizing on their market (for example the FCC in the US), but if successful this approach could disrupt the current 3GPP view on NTN.
While the R&D development are today focused on frequencies below 15 GHz, there is some interest in assessing achievable performances with a higher frequency (even if now standardization activity are for the moment ongoing either from 3GPP or WRC side). D band (~140 GHz) remains a strong topic of interest. For regulatory reasons, the 275 GHz – 325 GHz range holds promise as it enables the widest available bandwidth. As an illustration, the US has facilitated their research on the 95 GHz – 3 THz spectrum over the coming decade. After a unanimous vote, the Federal Communications Commission (FCC) has opened up the “terahertz wave” spectrum for experimental purposes, creating legal ways for companies to test and sell post-5G wireless equipment. However, we can note that the telecommunication industry is cautious on the use of this spectrum. Magnus Frodigh, Ericsson's chief researcher, for example wrote2: "We have thus identified new potential spectrum ranges for 6G, notably in the centimetric range from 7-15 GHz, which we believe will be an essential range, and in the sub-THz range from 92-300 GHz, which will have a complementary role serving niche scenarios". He continued: "Our learnings from 5G are that the mmWave range is a powerful spectrum range which allows operators to provide value to industry and enterprise through high data rates. However, due to limited coverage, it serves as a complement to other ranges that can be used in wider areas but with limited data rates (i.e. mid bands)." Frodigh concluded: "We believe that in order to benefit society, the majority of 6G use cases should be enabled for wide-area coverage, both indoors and outdoors, and not limited to confined areas. This means that – whilst we ought to explore the sub-THz region for entirely novel 6G capabilities – the main value will be in the centimetric 7-15 GHz". At such high frequency, new hardware technology may be required and InP is today a hot topic to enable low noise receivers and power efficient Power Amplifiers > 100 GHz.
Exploring the benefits that AI could bring to connectivity technologies
While 5G is being deployed around the world, efforts by both industry and academia have started to investigate beyond 5G to conceptualize 6G. 6G is expected to undergo an unprecedented transformation that will make it substantially different from the previous generations of wireless cellular systems. 6G may go beyond mobile internet and will be required to support ubiquitous AI services from the core to the end devices of the network. Meanwhile, AI will play a critical role in designing and optimizing 6G architectures, protocols and operations.
For example, two key 5G technologies are software-defined networking (SDN) and network functions virtualization (NFV), which have moved modern communications networks towards software-based virtual networks. As 6G networks are expected to be more complex and heterogeneous, advanced softwarisation solutions are needed for beyond 5G networks and 6G networks. Selecting the most suitable computational and network resources and the appropriate dynamic placement of network functions, taking network and application performance as well as power consumption and security requirements into account, will be an important topic. By enabling fast learning and adaptation, AI-based methods will render networks a lot more versatile in 6G systems. The design of the 6G architecture should follow an “AI-native” approach that will allow the network to be smart, agile, and able to learn and adapt itself according to changing network dynamics.
Given the integration of wireless and wired and legacy industrial edge connectivity, heterogeneous network virtualisation is of increasing importance to the System of Systems integration in all application domains. Manageable virtual heterogeneous networks from edge to cloud will become a strong competitive advancement in the near future. Given the foreseen network complexity AI assisted and partly autonomous management is clearly important.
To address identified connectivity technology challenges, we propose the vision described below, which can be summarized in the following three points (with associated expected outcomes).
Assess achievable connectivity performances using new spectrum
To maintain European leadership on connectivity technology and ensure sovereignty, the development of new electronics systems targeting connectivity applications in non-already standardized (or in the process of being standardized) spectrum should be supported. A special focus should be dedicated to the frequency bands listed below:
-
6G connectivity enablement in the 6.425 GHz – 7.125 GHz band: This band having been identified as the main one to be deployed at short terms for 6G, it is of strategic importance to ensure both sovereign European hardware development and a timely spectrum allocation to operator to secure the position of EU in the 6G race.
-
Investigation of additional 6G spectrum in the 7.75 GHz – 8.4 GHz band: This band being the most likely one at mid terms to support 6G deployment in the US, and also being a serious option to offer additional 6G spectrum worldwide, EU has to ensure a pragmatic evaluation of this spectrum to both be able to play a role in WRC body standardization activities and secure the market share of EU wireless infrastructure players on the US wireless market.
-
Investigation of Supplemental Connectivity from Space in existing 4G & 5G bands: While NTN in Ku and Ka band is today a hot topic inside 3GPP, it can not be excluded that SCS approach pushed by SpaceX and T-Mobile may disrupt current NTN view (in a very pragmatic way). Consequently, SCS scenario evaluation in EU leveraging existing cellular 4G & 5G spectrum should be encouraged and dedicated R&D activities supported to secure this technical option in EU and ensure both a EU sovereign connectivity solution and possible business opportunities for EU actors.
-
Investigate possible deployment of Wi-Fi-8 at 60 GHz: In the context of Wi-Fi-8 standard definition, Intel and other players have been pushing the support of the 60 GHz band to enable mmW based Wi-Fi connectivity (and achieved higher data rate than Wi-Fi-7 in a power efficient manner). The IEEE 802.11 Integrated Millimeter Wave Study Group (IMMW SG) has been specifically created in 2023 to investigate this scenario and decide about the addition of the 60 GHz band to Wi-Fi standard. R&D activity on this topic in EU should be encouraged to ensure that if selected this license free frequency band could be leveraged in EU to enable affordable high speed connectivity to the market.
-
Sub-THz connectivity application in D band (~140 GHz) and > 200 GHz: With THz communication being a hot topic in the international academic community (especially in the US and China), European activity in the spectrum > 200 GHz should be encouraged (with a special focus on high speed point to point wireless link). These investigations should help Europe play a role in the development of the new technology and assess its relevance for future standardization activities.
Integrate AI features to make connectivity technology faster, smarter and more power-efficient
The use of new spectrum or propagation mediums is not the only way to boost innovative connectivity technology. As mentioned, 5G has underlined the role of software to promote virtualization and reconfigurability, but those concepts may not be sufficient to address the challenges related to the more complex connectivity technology that may be developed (for example, 6G).
To address this challenge, Artificial Intelligence is now perceived as a strong enabler. Consequently, in coordination with the “Edge Computing and Embedded Artificial Intelligence” chapter, the topics below should be supported:
-
Investigate AI features at the edge: to improve the power efficiency of mobile devices by reducing the amount of data to be transmitted via the wireless network, the concept of AI at the edge (or edge AI) has been proposed. The idea is to locally process the data provided by the sensor using mobile device computing capability. Moreover, processing data locally avoids the problem of streaming and storing a lot of data to the cloud, which could create some vulnerabilities from a data privacy perspective.
-
Use AI to make the connectivity network more agile and efficient: the idea here is to move to an AI-empowered connectivity network to go beyond the concept of virtualization and achieve new improvements in terms of efficiency and adaptability. For example, AI could play a critical role in designing and optimizing 6G architectures, protocols and operations (e.g. resource management, power consumption, improved network performance etc.).
To support the vision presented in the previous paragraph, we propose that efforts should be focused on the following key focus areas:
-
Innovative connectivity system design using new spectrum.
-
Investigation and standardization activity targeting 6G cellular application in the frequency band < 15 GHz.
-
Development of innovative connectivity technology using unlicensed frequencies in the 6 GHz – 15 GHz band.
-
Development of connectivity system leveraging the concept of edge AI.
-
Evaluation of the AI concept to handle the complexity of future connectivity networks (for example, 6G), and to improve efficiency and adaptability.
2.2.3.3 Major Challenge 3: Autonomous interoperability translation for communication protocol, data encoding, compression, security and information semantics
Europe has a very clear technology lead in automation and digitalisation technology for industrial use. The next generation of automation technology is now being pushed by Industry 4.0/5.0 initiatives backed by the EC and most EU countries. In the automotive sector, the software defined vehicle initiative supports the autonomous and green car vision is the driver. Here, Europe again has a strong competitive position. In healthcare, the ageing population is the driver. Europe’s position in this area is respectable but fragmented. Robust, dependable, secure and interoperable connectivity from application to application and prepared for interaction in System of Systems solution are fundamental to market success in these and other areas.
Interoperability is a growing concern among numerous industrial players. An example here is the formation of industrial alliances and associated interoperability project efforts. One of the directions chosen targets is to gather behind a few large standards. An example of this is showcased in Figure 2.2.4.
To maintain and strengthen the European lead, advances in autonomous interoperability and associated efficient engineering capability are necessary. The game changers are:
-
Autonomous interoperability for SoS integration for efficient machine supported engineering at design-time and run-time.
-
Open interoperability infrastructure, and engineering support.
-
Novel, flexible and manageable security solutions.
-
Standardisation of the above technologies.

Figure 2.2.4 - ISO 15926 – Asset Standards worldwide (Source: Erik Molin, SEIIA)
To fully leverage heterogeneous integration at the hardware level, software interoperability is a parallel challenge to provide application to application connectivity that allows for autonomous SoS connectivity, from edge to cloud, enabling usage of available data for all areas of application. To do so, dedicated software tools, reference architecture infrastructure and standardisation are key to supporting autonomous interoperability, thus enabling the provision of a widely interoperable, secure, scalable, smart and evolvable SoS connectivity.
This challenge involves the interoperability of service or agent protocols, including encoding, security and data semantics. Here, payload semantics interoperability is a primary focus, leading to architectures, ontologies, technologies and engineering tools that support application to application integration of SoS for all areas of applications at design- time, in run-time and over life cycle. This will include e.g. integration of and translation between different standards used in domains where SoS interaction is necessary to reach business and societal objectives.
The objective here is a technology that enables nearly lossless interoperability across protocols, encodings and semantics, while providing technology and engineering support foundations for the low-cost integration of very large, complex and evolvable SoS.
Expected achievements are:
-
Open source SoS architecture infrastructure with reference implementation supporting interoperability, security scalability, smartness and evolvability across multiple technology platforms, including 5G & 6G.
-
Open source engineering and implementation infrastructure for the de-facto standard SoS connectivity architecture.
-
Architecture reference implementations with performance that meets critical performance requirements in focused application areas.
The high-priority technical and scientific challenges in both design-time and run-time are:
-
Semantics interoperability from application to application.
-
Autonomous translation of protocols, encodings, security and semantics.
-
Evolvable SoS connectivity architecture infrastructure and technologies over time and technology generations.
2.2.3.4 Major Challenge 4: Architectures and reference implementations of interoperable, secure, scalable, smart and evolvable IoT and SoS connectivity
It is clear that the US is the leader when it comes to wired IP-connectivity while Europe is the leader in cellular IP-connectivity. The big potential game changer here is 5G and upcoming 6G. To advance the European position, the establishment of connectivity architecture, reference implementation and associated engineering frameworks supporting 5G/6G and other wireless technologies is required. The integration of IP-connectivity with the vast industrial legacy edge connectivity needs a special focus. Such focus will foster a faster industrial and societal digitalisation enabling the integration of efficient and optimisable flexible production value networks. Given the longevity of such IP and legacy connectivity the life cycle perspective over technology life time and generations will need special considerations. In certain domains such as automotive and industrial automation, Europe is the major player. Market studies3 indicate very large to extreme growth in the SoS market over the next five to ten years. This will provide a very strong market pull for all technologies and products upstream. Here, connectivity interoperability is a very important component, enabling tailored SoS solutions and efficient engineering. The vision is to provide interoperable connectivity architecture, reference implementation and associated engineering support and frameworks spanning technologies from legacy to 5G and 6G and other wireless and wired technologies.
The enabling of SoS interoperable connectivity is fundamental for capturing the emerging SoS market and its very high growth rate. Efficient engineering and the deployment of interoperable, secure, scalable, smart and evolvable SoS connectivity will be key to this. This will help Europe lead in the establishment of interoperable connectivity architecture, reference implementation and associated engineering frameworks.
The identified game changers are:
-
Establishment of connectivity architecture infrastructure with associated reference implementation and related engineering frameworks and associated standardisation
-
SoS application to application connectivity being interoperable, secure, scalable, smart and evolvable over technology generations.
Expected achievements
-
Open source implementation of reference architecture infrastructure supporting interoperability, security scalability, smartness and evolvability across multiple technology platforms, like e.g. 5G/6G, wired and optical connectivity.
-
Open source engineering and implementation frameworks for the de-facto standard SoS connectivity architecture infrastructure.
-
Architecture infrastructure reference implementations which meet critical performance requirements in focused application areas.
The high-priority technical and scientific challenges are:
-
SoS interoperable connectivity architecture infrastructure as a de-facto standard.
-
Reference implementation of de-facto SoS connectivity architectures.
-
Engineering frameworks for de-facto standard SoS connectivity architecture.
2.2.3.5 Major Challenge 5: Network virtualisation enabling run-time engineering, deployment and management of edge to cloud network architectures.
Virtualisation of networks is a main trend for cellular networks. This has to be expanded to other wireless and wired connectivity technology including the vast industrial legacy edge connectivity. Current state of the art has a large technology differences between the legacy and IP based connectivity. This includes as well technology and operational competences in the industry and society. Thus wide and flexible digitalisation of industrial and societal value networks is rather limited.
The enabling of virtualised networks is fundamental for capturing the emerging SoS market and its very high growth rate. Efficient engineering, deployment and management of connectivity is a key enabler for interoperable, secure, scalable, smart and evolvable SoS. This will help Europe lead in the establishment of connectivity architecture, reference implementation and associated engineering frameworks.
European leadership in certain domains, mentioned above, will provide a very strong market pull for all technologies and products upstream. Here, virtualised connectivity is a very important component, enabling dynamic updates and rearrangements of SoS solutions. The vision is to provide virtualised connectivity across physical and mac layers spanning technologies from legacy to 5G and upcoming 6G.
The identified game changers are:
-
Technologies for network virtualisation across multiple hardware and software layers using heterogeneous devices.
-
Engineering, integration and management tools and methodologies for engineering and operation of virtualized legacy, IoT and SoS networks and service components of technology and system life cycle.
-
Intelligent and run-time re-organisation of hardware platforms.
Expected achievements:
-
Open source implementation of reference architectures supporting virtualised connectivity across multiple technology platforms from industry legacy to IP based 5G, 6G, wired and optical.
-
Open source engineering and management frameworks for virtualised connectivity across multiple technology platforms from industry legacy to IP based 5G, 6G, wired and optical.
-
Reference implementations with performance that meets critical performance requirements in focused application areas taking into consideration energy efficiency.
-
Run-time re-organisation of hardware and software platforms.
The high-priority technical and scientific challenges are:
-
Virtual connectivity architecture supporting multiple technology platforms.
-
Reference implementation of virtual connectivity architecture enabling very efficient application-level usage.
-
Engineering, integration and management frameworks with tools for virtual connectivity architectures.
The timeline for addressing the Major Challenges in this section is provided in the following table.
Major Challenge | Topic | Short Term (2025–2029) | Medium Term (2030–2034) | Long Term (2035 and beyond) |
---|---|---|---|---|
Major Challenge 1: Strengthening the EU connectivity technology portfolio to maintain leadership, secure sovereignty, and offer an independent supply chain |
Topic 1.1: Materials (GaN, InP, etc.) and large diameter wafers technology | TRL 3–4 Enable innovative GaN (on Si or on SiC), InP (on Si or on bulk InP) and POI technologies |
TRL 5–6 Industrial transfer of previous technologies from pilot line to Fab |
|
Topic 1.2: Differentiated semiconductor technology development targeting connectivity application | TRL 3–4 Enable next generation RF SOI and BiCMOS technology |
TRL 5–6 Industrial transfer of previous technologies from pilot line to Fab |
TRL 7–9 Support of high-volume production product in Europe |
|
Topic 1.3: Packaging and PCB technology targeting connectivity application | TRL 4–6 Development of innovative European packaging (such as AMP or FOWLP) and PCB technologies (targeting HDI) |
TRL 7–9 Pilot line enablement and support of small series |
||
Topic 1.4: Connectivity solution engineering through virtualization of the different connectivity layers | TRL 3–4 Proof of concept using existing COST hardware |
TRL 5–6 Dissemination through standardization bodies |
TRL 7–9 | |
Topic 1.5: Connectivity for high-speed low-energy heterogeneous and chiplet integration | TRL 3–4 Proof of concept at elementary function level (LNA, PA, VCO, mixer, …) leveraging pilot line available in Europe |
TRL 5–6 Higher complexity demo at the level of a full transceiver |
TRL 7–9 | |
Topic 1.6: Ultra-low power transceivers with low eco-footprint | TRL 4–6 Proof of concept at full transceiver level |
TRL 7–9 Transfer to the industry |
||
Major Challenge 2: Investigate innovative connectivity technology (new spectrum or medium) and new approaches to improving existing connectivity technology to maintain the EU’s long-term leadership |
Topic 2.1: Innovative connectivity system design using new spectrums | TRL 3–4 Assess the specification of wireless systems in new 6G band (< 7 GHz and prospective mmW band > 100 GHz) |
TRL 5–6 Achieve preliminary transceiver demonstrator using European technologies |
TRL 7–9 Transfer to the industry to enable European products |
Topic 2.2: Investigation and standardization activity targeting 6G cellular application in frequency band < 10 GHz | TRL 3–4 Contribute to 6G new spectrum standardization (especially the 7.1 GHz – 8.4 GHz band targeted by WRC27) |
TRL 5–6 Leverage European differentiated technologies to address 6G mid-band spectrum challenges |
TRL 7–9 Enable first 6G connectivity chipset solution |
|
Topic 2.3: Development of innovative connectivity technology using unlicensed frequency in the 6 GHz – 7 GHz and 60 GHz band | TRL 4–6 Contribute to Wi-Fi8 standardization (especially on low power and Integrated Millimeter Wave features) |
TRL 7–9 Enablement of European Wi-Fi 7 and 8 chipset solution leveraging European derivative technology |
||
Topic 2.4: Development of connectivity systems leveraging the concept of edge AI | TRL 3–4 Proof of concept using existing COST hardware |
TRL 5–6 Dissemination through standardization bodies |
||
Topic 2.5: Evaluation of the AI concept to handle the complexity of future connectivity networks (e.g., 6G) | TRL 3–4 Proof of concept using existing COST hardware |
TRL 5–6 Dissemination through standardization bodies |
||
Major Challenge 3: Autonomous interoperability translation for communication protocol, data encoding, compression, security, and information semantics |
Topic 3.1: Semantics interoperability from application to application | AI-supported translation of payload semantics based on limited ontologies and standards | Scalability to payload semantics integration across domains | Scalability to payload semantics integration between application domains |
Topic 3.2: Autonomous translation of protocols, encodings, security, and semantics | Autonomous dynamic translation between SOA-based protocols, encoding, compression, and encryption | Dynamic translation across major data models relevant for ECS | Dynamic translation across a large set of data models relevant for ECS | |
Topic 3.3: Evolvable SoS connectivity architecture infrastructure | AI-supported evolution of connectivity infrastructure over time | Formal tools to support evolution of connectivity infrastructure | AI-supported V&V of evolving connectivity infrastructure | |
Major Challenge 4: Architectures and reference implementations of interoperable, secure, scalable, smart, and evolvable IoT and SoS connectivity |
Topic 4.1: SoS interoperable connectivity architecture | Established as a major industrial choice within ECS application domains | Established as a major industrial choice across ECS domains | Established as a major industrial and societal choice |
Topic 4.2: Reference implementation of SoS connectivity architecture | Natural part of global SoS architecture (chapter SoS) reference implementation | TRL 8–9: Reference implementation of global SoS architecture | Including semantic data model interoperability | |
Topic 4.3: Engineering frameworks for SoS connectivity architecture | Engineering framework with tools for SoS interoperable connectivity | Standardized framework with associated tools | Standardized framework including wide data model translation | |
Major Challenge 5: Network virtualization enabling runtime engineering, integration, deployment, and management of edge and cloud network architectures |
Topic 5.1: Virtual connectivity architecture supporting multiple technology platforms | Distributed edge-to-cloud virtual connectivity environment with limited platforms | Including legacy and IP-based technology platforms | Across legacy and IP-based platforms |
Topic 5.2: Reference implementation of virtual connectivity architecture | Integration of connectivity technology platforms supporting virtualization | Pilot demonstrations with accelerators lowering cost significantly | Pilot demonstrations covering legacy to IP-based platforms | |
Topic 5.3: Engineering, integration, and management frameworks | Virtualization engineering tools for edge-to-cloud integration | Pilot-scale toolchains for edge-to-cloud integration | Full virtualization engineering across legacy and IP-based platforms |